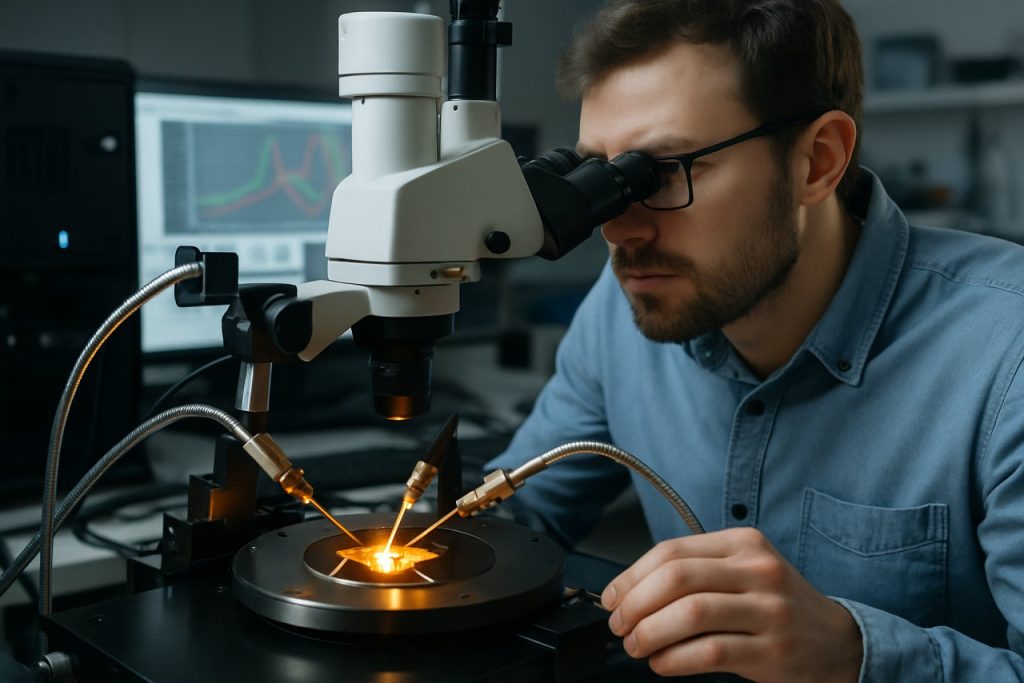
Table of Contents
- Executive Summary & Key Findings for 2025–2030
- Technology Landscape: Cutting-Edge Techniques in Excitonic Materials Analysis
- Market Size, Growth Forecasts & Emerging Trends (2025–2030)
- Leading Players & Recent Strategic Developments
- End-User Segments: Electronics, Photonics, Energy & Beyond
- Breakthroughs in Instrumentation & Analytical Protocols
- Regional Analysis: Hotspots for Innovation & Investment
- Partnerships, Collaborations & Industry Initiatives
- Challenges: Technical Barriers, Standardization & Regulatory Outlook
- Future Outlook: Disruptive Opportunities and Market Predictions
- Sources & References
Executive Summary & Key Findings for 2025–2030
The market for excitonic materials characterization services is entering a pivotal growth phase between 2025 and 2030, driven by advances in quantum materials, optoelectronics, and next-generation photovoltaics. Excitonic materials—where electron-hole pairs (excitons) dominate optical and electronic properties—are central to the development of high-performance light-harvesting, quantum information, and display technologies. The demand for sophisticated characterization services is escalating as research and commercial efforts intensify in perovskite solar cells, transition metal dichalcogenides (TMDs), quantum dots, and organic semiconductors.
Key service providers are expanding their capabilities to address the technical challenges of excitonic phenomena, including ultrafast spectroscopy, spatially resolved photoluminescence, and advanced microscopy. Major instrument manufacturers such as Bruker and Olympus Corporation continue to innovate in spectroscopy and imaging platforms tailored for nanoscale and time-resolved studies necessary for excitonic systems. Additionally, specialized providers like Oxford Instruments are integrating cryogenic measurement solutions to probe exciton dynamics at low temperatures, which is crucial for quantum device development.
Recent industry events highlight a trend towards collaborative service models, where analytical laboratories partner with academic and industrial research centers to accelerate material validation and device prototyping. The growing participation of companies such as Thermo Fisher Scientific in offering contract materials analysis and custom spectroscopy solutions underscores the sector’s shift to flexible, project-based support. Moreover, the convergence of AI-driven data analytics with high-throughput characterization, as seen in initiatives by Agilent Technologies, is expected to further enhance the speed and accuracy of excitonic materials evaluation.
Looking ahead to 2030, key findings suggest that the excitonic materials characterization services market will be shaped by:
- Continued investment in advanced instrumentation for ultrafast and multidimensional spectroscopy.
- Increasing integration of cryogenic and in situ measurement capabilities to support quantum and optoelectronic applications.
- Expansion of tailored contract services and collaborative platforms between equipment suppliers, CROs, and research institutions.
- Adoption of data-driven approaches, leveraging machine learning and automation to accelerate analysis and material discovery.
Overall, the period from 2025 to 2030 is set to witness robust growth in excitonic materials characterization services, propelled by technological innovation and a maturing ecosystem of providers and customers across the semiconductor, energy, and quantum technology sectors.
Technology Landscape: Cutting-Edge Techniques in Excitonic Materials Analysis
The field of excitonic materials characterization is witnessing rapid technological advancement, driven by demand for high-performance optoelectronic devices, photonics, and quantum information systems. As of 2025, service providers and research institutes are leveraging a suite of advanced analytical techniques to probe excitonic phenomena—such as binding energies, lifetimes, diffusion behaviors, and coupling effects—at the nanometer and femtosecond scales.
Time-resolved photoluminescence (TRPL) and ultrafast pump-probe spectroscopy remain foundational for characterizing exciton dynamics, providing sub-picosecond temporal resolution critical for understanding recombination processes in materials like transition metal dichalcogenides (TMDs) and perovskites. Cryogenic microscopy and spectroscopy, including micro-PL and scanning near-field optical microscopy (SNOM), are increasingly adopted to spatially map excitonic states in low-dimensional materials. Providers such as Bruker and Oxford Instruments continue to enhance their instrument platforms to cater to these needs, integrating low-temperature and high-magnetic-field capabilities.
Emerging techniques in 2025 include multidimensional coherent spectroscopy, which enables the disentanglement of coupled electronic and vibrational states, and tip-enhanced Raman spectroscopy (TERS) for nanoscale mapping of excitonic features. These are being rapidly integrated into service offerings by specialized labs and instrument manufacturers. For example, HORIBA is expanding its spectroscopy solutions to support increasingly complex excitonic measurements, reflecting growing client demand from semiconductor and quantum technology sectors.
Another notable development is the rise of in situ and operando characterization, allowing real-time monitoring of excitonic behavior under device-relevant conditions such as electrical biasing or illumination. This is particularly relevant for industrial research, where companies like Thermo Fisher Scientific have introduced integrated platforms that combine electron microscopy with in situ optical and electrical probing.
Looking ahead, the landscape is expected to further evolve through the adoption of artificial intelligence and machine learning algorithms for data analysis and interpretation, enabling automated extraction of excitonic parameters from complex multidimensional datasets. Standardization efforts by industry bodies are also anticipated to foster interoperability and reliability among characterization service providers. As demand grows from sectors such as next-generation photovoltaics, light-emitting devices, and quantum computing, the scope and sophistication of excitonic materials characterization services will continue to expand through 2025 and beyond.
Market Size, Growth Forecasts & Emerging Trends (2025–2030)
The market for excitonic materials characterization services is entering a dynamic growth phase as demand surges from advanced materials research, optoelectronics, and quantum technologies sectors. In 2025, the global landscape is defined by an uptick in public and private investment targeting the development of perovskite photovoltaics, organic LEDs, and next-generation semiconductors—all of which require precise characterization of excitonic behaviors to optimize device performance. This trend is driving both established analytical instrumentation firms and specialized service providers to expand their capabilities.
Throughout 2025, the sector is expected to exhibit robust growth, with key players such as Bruker, Oxford Instruments, and HORIBA actively enhancing their service portfolios to offer advanced photoluminescence, time-resolved spectroscopy, and ultrafast pump-probe analyses. These companies are investing in new infrastructure and digital platforms to facilitate remote data access and collaborative interpretation, responding to a growing trend of distributed R&D and the globalization of innovation networks.
Academic research centers and national laboratories are also increasing outsourcing of advanced characterization tasks to external service providers, catalyzed by rapid developments in 2D materials and hybrid organic-inorganic systems. For instance, the expansion of services at institutions like National Institute of Standards and Technology and partnerships with industry are enabling broader access to state-of-the-art measurement techniques, including exciton diffusion mapping and quantum yield estimation.
Looking ahead to 2030, several emerging trends are poised to shape the market. First, the adoption of machine learning and AI-driven interpretation tools is anticipated to accelerate the throughput and accuracy of complex excitonic measurements. Second, sustainability considerations are prompting the development of characterization protocols tailored to eco-friendly materials and low-energy device architectures. Third, the proliferation of quantum computing and communication research is expected to drive demand for characterization services addressing quantum-confined excitonic phenomena.
Geographically, Asia-Pacific is forecast to register the fastest growth in demand for excitonic materials characterization services, propelled by aggressive investments in semiconductor and display technologies from countries including China, South Korea, and Japan. Meanwhile, North America and Europe continue to emphasize integration of service offerings with broader R&D ecosystems, fostering innovation through cross-disciplinary collaborations and digital workflow integration.
As the field matures, industry leaders and new entrants alike are pursuing standardization initiatives—often in collaboration with organizations such as Semiconductor Industry Association—to ensure data comparability, reproducibility, and regulatory compliance. These efforts are expected to reduce barriers to adoption and support sustained market expansion through 2030.
Leading Players & Recent Strategic Developments
The landscape of excitonic materials characterization services in 2025 is defined by the participation of advanced instrumentation companies, integrated research centers, and key suppliers focusing on next-generation optoelectronic materials. As the demand for precision analysis of excitonic phenomena in materials such as transition metal dichalcogenides (TMDs), perovskites, and organic semiconductors accelerates, several organizations have taken strategic steps to expand capabilities and global reach.
Among the market leaders, Bruker Corporation continues to push forward with upgrades to its spectroscopy and microscopy platforms, enabling more refined studies of exciton dynamics and nanoscale interactions. In 2024–2025, Bruker has expanded its service offerings to accommodate ultrafast spectroscopy and advanced photoluminescence mapping, specifically targeting industrial and academic clients working on excitonic materials.
Meanwhile, Oxford Instruments has strengthened its role in workflow integration for excitonic materials analysis by combining cryogenic systems and high-resolution microscopy tools. Recent collaborations with research clusters and semiconductor manufacturers in Europe and Asia have allowed Oxford Instruments to deliver tailored solutions for atomically thin materials and hybrid perovskites characterization—a trend expected to intensify as new device applications emerge.
On the analytical front, Thermo Fisher Scientific expanded its electron microscopy and spectrometry services in 2025, supporting contract research and industrial labs worldwide. Their latest developments focus on correlating structural and excitonic properties at the atomic scale, a capability that has become critical for clients in the quantum materials and advanced photovoltaics sectors.
Strategic partnerships and infrastructure investments are shaping the sector’s trajectory. For instance, HORIBA Scientific has introduced new modular solutions integrating time-resolved photoluminescence and Raman spectroscopy, with turnkey service models aimed at startups and research institutes entering the excitonic materials space. Additionally, JEOL Ltd. has advanced its support for ultrafast electron microscopy and cathodoluminescence, key techniques for visualizing excitonic effects in low-dimensional materials.
Looking ahead, the next few years are expected to see further consolidation among service providers and deeper collaboration with semiconductor and display manufacturers. As demand rises for advanced characterization of excitonic materials—especially for quantum computing, photonics, and flexible electronics—leading companies are anticipated to invest in automation, AI-powered data analysis, and cross-disciplinary partnerships to maintain a competitive edge.
End-User Segments: Electronics, Photonics, Energy & Beyond
Excitonic materials, which leverage electron-hole pair interactions, are increasingly critical across multiple high-technology sectors. In 2025 and the coming years, the demand for advanced characterization services for excitonic materials is set to rise, driven by rapid innovation in electronics, photonics, and energy applications. Key end-user segments include manufacturers of optoelectronic devices, solar cells, light-emitting diodes (LEDs), and emerging quantum technologies.
In the electronics industry, the integration of excitonic materials into ultra-thin transistors and next-generation displays is creating a need for precise structural, optical, and electronic property analysis. Companies such as Samsung Electronics and LG Electronics have announced R&D initiatives focusing on materials like transition metal dichalcogenides (TMDs) and perovskites, which require meticulous characterization to optimize charge carrier dynamics and device stability.
Photonics is another rapidly evolving end-use sector. Here, excitonic effects are fundamental to the development of high-efficiency lasers, single-photon emitters, and photodetectors. Characterization services are essential for mapping exciton diffusion, recombination rates, and coherence properties. Organizations such as Hamamatsu Photonics and Coherent are actively developing and utilizing advanced spectroscopy and microscopy tools tailored for these tasks, enabling better understanding of excitonic behavior at the nanoscale.
In the energy segment, the push for next-generation solar cells—especially using perovskite and organic photovoltaic materials—relies heavily on detailed excitonic characterization. Companies like First Solar and Oxford PV are scaling up new solar modules where understanding exciton dissociation and energy transfer is crucial for efficiency improvements and reliability. These organizations often collaborate with specialized materials analysis providers for advanced photoluminescence, transient absorption, and electron microscopy services.
Beyond traditional electronics and energy, emerging areas such as quantum computing and neuromorphic hardware are creating new opportunities for excitonic materials characterization. As commercial players and research institutions accelerate efforts in quantum photonics, the demand intensifies for services capable of probing ultrafast excitonic processes and coherence lifetimes.
Looking forward, the outlook for excitonic materials characterization services is robust, supported by increased investment in R&D and a growing ecosystem of manufacturers and end-users. As device architectures become more complex and performance requirements more stringent, the role of precise and application-specific characterization will only expand, underpinning advances across electronics, photonics, energy, and beyond.
Breakthroughs in Instrumentation & Analytical Protocols
Recent years have witnessed significant advancements in the instrumentation and analytical protocols deployed within excitonic materials characterization services. As the demand for high-efficiency optoelectronic devices and next-generation solar cells intensifies, service providers and equipment manufacturers are rapidly innovating to offer more precise and comprehensive characterization of excitonic phenomena.
Key breakthroughs in 2025 include the integration of ultrafast spectroscopy systems capable of resolving exciton dynamics on femtosecond timescales, which is crucial for understanding the performance limits of materials such as perovskites, organic semiconductors, and quantum dots. Companies like Bruker Corporation and HORIBA are now supplying modular spectrometers and time-correlated single-photon counting (TCSPC) systems with enhanced sensitivity, enabling service labs to extract detailed information on exciton lifetimes and migration pathways with unprecedented clarity.
Additionally, developments in spatially resolved photoluminescence (PL) mapping and hyperspectral imaging are allowing researchers to directly visualize excitonic effects across device-relevant length scales. For instance, Oxford Instruments continues to refine its suite of cryogenic microscopy platforms, facilitating temperature-dependent studies and correlative analyses that link excitonic behavior to material microstructure. The adoption of automated data acquisition and machine learning-driven data analysis is also gaining momentum, further enhancing throughput and reproducibility for contract characterization services.
Another notable trend is the emergence of combined in situ and operando measurement capabilities. Service providers and instrument manufacturers are equipping systems to probe excitonic states under applied bias or operating conditions, providing insights directly relevant to device performance and stability. Advancements in ultrafast transient absorption and time-resolved electron microscopy, as developed by firms like JEOL Ltd., are expanding the analytical toolkit available for probing excitonic processes in real time and under realistic environmental stimuli.
Looking ahead, the outlook for excitonic materials characterization services remains robust. Demand is set to rise as industries ranging from photovoltaics to quantum computing accelerate materials development pipelines. Service labs are expected to further integrate multi-modal analytical suites and advanced automation, minimizing turnaround times while maximizing data fidelity. As instrumentation capabilities mature, the focus will likely shift toward the standardization of protocols and interoperability between platforms, fostering greater collaboration between instrument makers, service providers, and end-users.
Regional Analysis: Hotspots for Innovation & Investment
The global landscape for excitonic materials characterization services is experiencing rapid evolution in 2025, with pronounced regional hotspots emerging due to concentrated R&D activity, government incentives, and robust industry-academic collaboration. Key regions such as North America, Europe, and East Asia are leading innovation and investment, driven by their advanced semiconductor, optoelectronics, and quantum materials sectors.
In North America, the United States maintains its leadership through a dense network of research universities, national laboratories, and advanced instrumentation firms. The region benefits from public–private initiatives and funding, particularly in quantum technologies and next-generation photovoltaics, where excitonic materials are crucial. Companies such as Bruker and Thermo Fisher Scientific offer state-of-the-art spectroscopy and microscopy solutions tailored for nanoscale excitonic property analysis, supporting both academic and industrial clients. Canada, meanwhile, leverages its materials science research base and government-backed innovation clusters, notably in Ontario and Quebec, to foster start-ups and scale-ups specializing in novel characterization techniques.
Europe continues to be a significant hub, anchored by Germany, the United Kingdom, and France. The European Union’s focus on digital sovereignty, renewable energy, and advanced manufacturing underpins substantial investments in characterization infrastructure and collaborative research. Entities like Carl Zeiss AG and Oxford Instruments provide comprehensive platforms for excitonic materials analysis, including advanced photoluminescence and ultrafast spectroscopy systems. National initiatives such as Germany’s High-Tech Strategy and the UK’s Catapult centres are catalyzing partnerships between universities and industry, with a particular emphasis on scaling quantum and organic electronic technologies.
East Asia, led by Japan, South Korea, and China, is rapidly expanding its capabilities. Japan’s established electronics sector and materials science prowess, exemplified by companies like HORIBA, foster continuous innovation in excitonic materials testing. South Korea’s strategic investments in semiconductor R&D and China’s focus on domestic high-tech manufacturing are resulting in new facilities and talent pipelines for advanced characterization services. Regional governments are supporting these efforts with funding programs and public–private partnerships, accelerating commercialization timelines.
Looking ahead, emerging markets in Southeast Asia and India are poised to increase their profile as local universities and technology parks gain access to advanced instrumentation and global collaboration networks. Overall, the next few years will see intensified competition and cross-border partnerships, as regions vie for leadership in the commercialization of excitonic materials for quantum, optoelectronic, and energy applications.
Partnerships, Collaborations & Industry Initiatives
The field of excitonic materials characterization services is witnessing an accelerated formation of partnerships and collaborative initiatives as industry and academia strive to unlock the potential of next-generation optoelectronic and quantum devices. In 2025, the momentum is particularly strong among companies, research institutes, and instrumentation manufacturers, motivated by the need for advanced analytical capabilities to probe excitonic phenomena in novel materials such as perovskites, 2D semiconductors, and organic crystals.
Key players in scientific instrumentation, such as Bruker and Oxford Instruments, are expanding their ecosystems through collaborations with leading universities and industrial partners. These collaborations are aimed at co-developing and refining characterization techniques—including time-resolved photoluminescence, transient absorption spectroscopy, and ultrafast microscopy—tailored for exciton dynamics and binding energy measurements. For instance, Bruker is known to engage with global research consortia to enhance the sensitivity and resolution of their spectroscopic systems for the study of low-dimensional materials.
Another notable trend in 2025 is the emergence of dedicated service providers and contract research organizations specializing in advanced excitonic materials analysis. Companies like Eurofins EAG Laboratories are extending their offerings to include custom characterization support, leveraging their expertise in electron microscopy, atomic force microscopy, and related techniques that provide insight into excitonic behavior at the nanoscale.
Industry-led initiatives are also being supported by national and regional innovation programs. For example, the European Union’s ongoing funding of collaborative research projects involving leading materials science centers and private sector partners is fostering the development of standardized protocols and interoperable data platforms for excitonic materials testing. Further, major semiconductor and display manufacturers—including Samsung Electronics and LG Electronics—are partnering with instrumentation specialists and academic consortia to accelerate the commercialization of excitonic materials for applications in displays, photovoltaics, and quantum information technologies.
Looking ahead, these partnerships are expected to deepen as the complexity of excitonic materials increases and the demand for reliable, high-throughput characterization grows. The next few years will likely see tighter integration between hardware developers, service providers, and end-users, with an emphasis on open innovation platforms and shared infrastructure. Such collaborative efforts are poised to drive advancements in measurement standards, reproducibility, and data sharing—critical enablers for the broader adoption of excitonic materials in commercial technologies.
Challenges: Technical Barriers, Standardization & Regulatory Outlook
The rapid evolution of excitonic materials—central to next-generation optoelectronic devices, quantum computing elements, and advanced sensors—demands sophisticated characterization services. However, technical, standardization, and regulatory challenges persist as the sector matures in 2025 and beyond.
A primary technical barrier lies in the intrinsic complexity of excitonic phenomena, which often occur at the nanoscale and under specific environmental conditions (e.g., low temperatures, high vacuum, or in the presence of strong electromagnetic fields). As a result, advanced instrumentation such as ultrafast spectroscopy, cryogenic microscopy, and time-resolved photoluminescence are essential for accurate characterization. The precision and reproducibility required have led to an increased demand for instrument calibration, operator expertise, and sophisticated data analysis methodologies. Even leading providers, such as Bruker and Oxford Instruments, face ongoing R&D hurdles in adapting their systems to the unique needs of excitonic materials, including atomic layer heterostructures and organic-inorganic perovskites.
Standardization presents a further challenge. As the market for excitonic materials characterization grows, so does the need for globally recognized protocols and benchmarks. The absence of unified standards for sample preparation, measurement conditions, and data interpretation leads to discrepancies between labs and hampers cross-comparison of results. Organizations such as the ASTM International and International Organization for Standardization (ISO) have begun preliminary work on standardizing optical and electronic property measurements for novel materials, but comprehensive standards tailored to excitonic phenomena remain under development. Industry consortia and academic collaborations are expected to accelerate this process over the next several years, fostering interoperability and more reliable quality control.
On the regulatory front, the outlook is cautiously optimistic. Regulatory frameworks governing advanced materials are evolving, with a focus on environmental impact, material safety, and device reliability. For companies offering characterization services, demonstrating compliance with emerging regulations—such as the European Union’s REACH and RoHS directives, and similar initiatives in North America and Asia—will be increasingly important, particularly as excitonic materials move into commercial applications. Service providers are investing in traceability, documentation, and robust quality management systems to align with these requirements.
Looking ahead, overcoming these challenges will require sustained investment in metrology R&D, closer alignment between industry and standards bodies, and proactive engagement with regulators. The sector’s ability to address these barriers will determine how quickly excitonic materials can transition from laboratory curiosities to commercially viable technologies.
Future Outlook: Disruptive Opportunities and Market Predictions
Looking ahead to 2025 and beyond, the future of excitonic materials characterization services is poised for significant transformation, driven by converging advances in spectroscopy, computation, and industrial demand for next-generation optoelectronics. Excitonic materials—where electron-hole pairs strongly interact—are at the center of innovations in solar energy, photodetectors, quantum computing, and displays. As these materials move from research labs to industrial application, the demand for precise, rapid, and scalable characterization services is expected to surge.
In 2025, leading characterization equipment providers and specialized service labs are expected to expand their portfolios to address the unique requirements of excitonic materials. This includes advanced ultrafast spectroscopy, time-resolved photoluminescence, and nanoscale imaging modalities, leveraging recent improvements in detector sensitivity and data analytics. Companies such as Bruker and Oxford Instruments are already innovating in high-resolution microscopy and spectroscopy systems suited for excitonic research, and are likely to integrate AI-driven automation and remote analysis to meet growing throughput demands.
The outlook is further shaped by the rise of quantum technologies and two-dimensional (2D) materials like transition metal dichalcogenides, where excitonic effects dominate device performance. The pace of commercialization in these sectors will necessitate third-party characterization labs to offer highly standardized, reproducible, and rapid turnaround services. Industry bodies such as SEMI are expected to play a role in defining best practices and data standards, as collaborative consortia between equipment manufacturers, material suppliers, and end-users become more prevalent.
In the next few years, disruptive opportunities are anticipated from the integration of in situ and operando characterization—enabling real-time monitoring of excitonic behavior during device fabrication or under operational stress. This is likely to be supported by instrument makers including HORIBA and Thermo Fisher Scientific, whose modular spectroscopy and microscopy platforms are being adapted for such advanced workflows. Furthermore, cloud-based data sharing and AI-assisted data interpretation will increasingly be bundled with service offerings, lowering the barrier for small- and medium-sized enterprises to access cutting-edge analysis.
By 2028, the global market for excitonic materials characterization services is projected to mature, with standardization, automation, and digital integration defining competitive advantage. Companies that can offer scalable, interoperable, and rapid characterization—while supporting the transition from fundamental research to high-volume manufacturing—are positioned to capture significant value in the evolving optoelectronics and quantum materials ecosystem.
Sources & References
- Bruker
- Olympus Corporation
- Oxford Instruments
- Thermo Fisher Scientific
- HORIBA
- National Institute of Standards and Technology
- Semiconductor Industry Association
- JEOL Ltd.
- LG Electronics
- Hamamatsu Photonics
- Coherent
- First Solar
- Oxford PV
- Bruker
- Thermo Fisher Scientific
- Carl Zeiss AG
- Oxford Instruments
- HORIBA
- Eurofins EAG Laboratories
- ASTM International
- International Organization for Standardization (ISO)