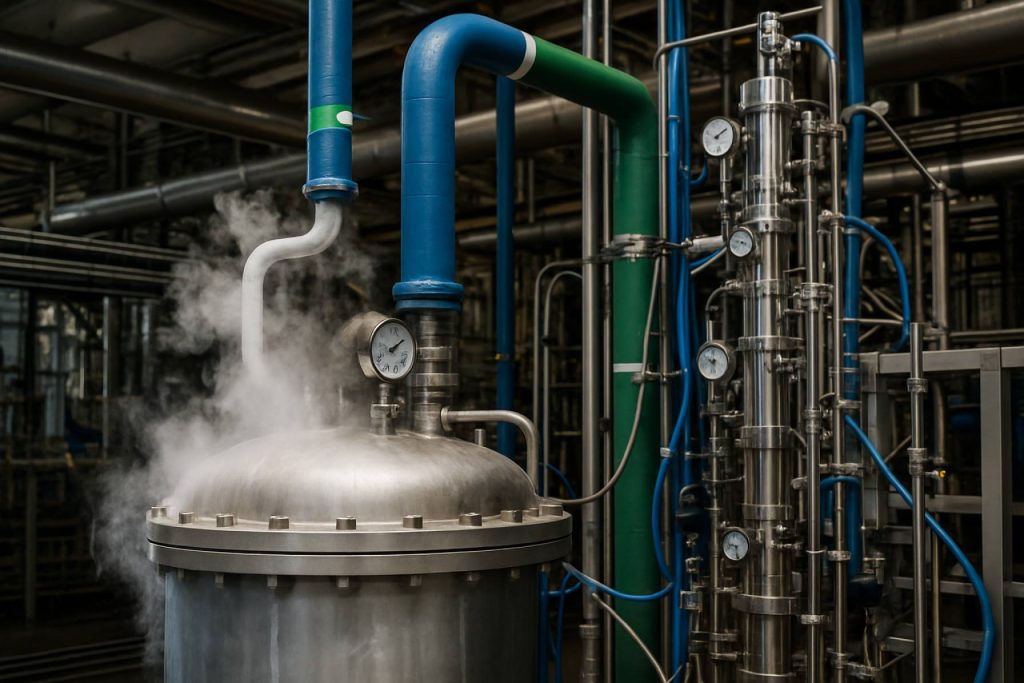
Table of Contents
- Executive Summary: Key Trends in Cryogenic Isotopic Separation (2025–2030)
- Market Size and Forecast: Global and Regional Growth Projections
- Emerging Applications: Clean Energy, Nuclear, and Quantum Technologies
- Competitive Landscape: Leading Players and Recent Strategic Moves
- Technological Innovations: Cryogenic Techniques and System Advancements
- Supply Chain Dynamics and Raw Material Considerations
- Regulatory Environment and International Standards
- Challenges: Technical, Economic, and Environmental Barriers
- Investment and Partnership Opportunities in 2025 and Beyond
- Future Outlook: Disruptive Trends and Long-Term Market Potential
- Sources & References
Executive Summary: Key Trends in Cryogenic Isotopic Separation (2025–2030)
Cryogenic isotopic separation, a critical technology for producing high-purity isotopes for nuclear, medical, and industrial applications, is set for significant transformation between 2025 and 2030. The sector is witnessing a convergence of innovation, policy momentum, and growing market demand, especially as global interest in advanced nuclear reactors, fusion research, and radioisotope production intensifies.
One of the most prominent trends is the advancement of cryogenic distillation and rectification systems, particularly for the separation of hydrogen and oxygen isotopes. Major players such as Linde and Air Liquide continue to invest in optimizing cryogenic processes that improve isotope yield, reduce energy consumption, and enhance operational safety. These innovations are driven by the need for enriched deuterium and tritium—essential for fusion power projects such as ITER and future commercial fusion plants.
The demand for stable isotopes, notably 18O, 15N, and 13C, is also rising sharply, fueled by their use in medical diagnostics (PET imaging), pharmaceuticals, and environmental tracing. Cryogenic methods remain the gold standard for large-scale oxygen isotope enrichment, with suppliers like Isoflex USA and Campro Scientific meeting growing orders from the life sciences and energy sectors.
A key development is the integration of digital process controls and real-time analytical technologies, enabling finer process tuning and improved product quality. The implementation of Industry 4.0 technologies is expected to be widespread by 2030, further boosting efficiency and traceability across production lines.
Geopolitical and supply chain considerations are shaping the industry’s outlook as well. The push for domestic isotope production in North America, Europe, and East Asia is accelerating investments in new cryogenic separation facilities, aiming to reduce reliance on imports and address strategic vulnerabilities. For instance, Rosatom and Orano are expanding their capabilities to serve both internal and export markets, while partnerships with fusion and medical research organizations are becoming more common.
Looking ahead to 2030, the cryogenic isotopic separation sector is expected to benefit from sustained R&D, increased automation, and greater integration with downstream applications. Market growth will be most pronounced in regions investing in fusion energy and advanced nuclear medicine, positioning cryogenic separation as a cornerstone technology for the next wave of scientific and industrial progress.
Market Size and Forecast: Global and Regional Growth Projections
Cryogenic isotopic separation, leveraging the distinct boiling points of isotopes—most notably in the separation of oxygen, nitrogen, and hydrogen isotopes—remains critical for industries spanning nuclear energy, medical diagnostics, and advanced materials. As of 2025, the global market for cryogenic isotopic separation is closely tied to the expansion of nuclear power generation, rising demand for stable isotopes in medicine and research, and government initiatives aimed at ensuring secure isotope supply chains.
In 2025, the global market size for cryogenic isotopic separation is estimated to be in the low-to-mid single billion USD range, with significant contributions from regions with robust nuclear and medical infrastructure, such as North America, Europe, and parts of Asia-Pacific. Industry leaders, including Air Liquide and Linde plc, continue to invest in cryogenic separation technologies for both bulk gas production and high-purity isotope extraction. This market is expected to grow at a compound annual growth rate (CAGR) in the high single digits through the late 2020s, driven by expansion in energy and healthcare sectors.
Regionally, North America remains a key market, propelled by the United States’ ongoing investment in uranium enrichment and tritium production for both civilian and defense purposes. The U.S. Department of Energy’s continued support for domestic isotope production and enrichment infrastructure is expected to maintain momentum in this sector. In Europe, investments are being made to modernize and expand cryogenic separation capacity, particularly in France and Germany, to support both nuclear and medical isotope needs, with companies like Air Liquide leading these initiatives.
The Asia-Pacific region, led by China, Japan, and South Korea, is forecasted to demonstrate the fastest market growth through 2028. This expansion is underpinned by aggressive nuclear energy strategies and emerging domestic capabilities in isotope production. For example, Air Liquide and Linde plc have both expanded their operations and partnerships in the region to support growing demand.
Looking ahead, market growth will be further supported by technological advancements that increase process efficiency and output purity. Strategic collaborations between industrial gas companies and research institutions are expected to accelerate deployment of next-generation cryogenic separation plants. However, the sector remains sensitive to geopolitical developments, regulatory frameworks, and the pace of nuclear energy adoption globally. Overall, the outlook for cryogenic isotopic separation is positive, with robust demand anticipated from both established and emerging markets in the next several years.
Emerging Applications: Clean Energy, Nuclear, and Quantum Technologies
Cryogenic isotopic separation, a process leveraging extremely low temperatures to distinguish isotopes based on minute differences in their physical properties, is gaining renewed relevance across multiple high-impact sectors as of 2025. Historically crucial for nuclear fuel enrichment, the technology now stands at the intersection of clean energy, advanced nuclear systems, and the rapidly evolving quantum technology landscape.
In the clean energy domain, cryogenic separation is increasingly applied to the production of isotopically enriched materials—chiefly deuterium and tritium—for use in fusion energy research. The global race to realize commercial fusion reactors, led by international projects and private sector investments, demands large-scale, high-purity deuterium and tritium as fusion fuels. Cryogenic distillation is the preferred technique for heavy water (D2O) and tritium recovery due to its scalability and high selectivity. Firms with expertise in this area, such as Linde and Air Liquide, are actively developing and deploying advanced cryogenic systems in support of fusion pilot plants and experimental facilities worldwide.
For the nuclear sector, cryogenic methods are being refined to support next-generation reactor designs that require specific isotopes with enhanced neutron economy, such as uranium-235 or boron-10. The ability to efficiently separate and purify these isotopes is crucial for the operation of advanced reactors, medical isotope production, and for fuel cycle optimization. Companies offering cryogenic solutions and custom gas separation systems, such as Praxair (now part of Linde), are well-positioned to meet the anticipated uptick in demand as new nuclear projects transition from demonstration to deployment.
Quantum technology is another frontier driving innovation in cryogenic isotopic separation. Isotopically pure silicon-28 and diamond (carbon-12) are foundational materials for quantum computing and ultra-sensitive sensors, as they minimize decoherence in quantum bits (qubits). The production of such ultrapure materials relies on advanced cryogenic separation steps for precursor gases, a need recognized by materials and specialty gas suppliers working closely with quantum hardware firms. As quantum computing moves toward commercialization, the demand for such isotopically tailored inputs is expected to grow markedly.
Looking ahead to the next several years, industry experts anticipate that advances in cryogenic engineering, automation, and process intensification will drive down costs and increase throughput, making isotopic separation more accessible across these emerging sectors. Strategic partnerships between industrial gas companies and clean energy or quantum technology developers are expected to accelerate the deployment of scalable cryogenic separation infrastructure worldwide.
Competitive Landscape: Leading Players and Recent Strategic Moves
The competitive landscape of cryogenic isotopic separation in 2025 is defined by a small cohort of global leaders, specialized technology providers, and strategic collaborations responding to rising demands in nuclear, healthcare, and research sectors. The technology is capital-intensive and highly regulated, resulting in prominent positions for established industrial gas giants and government-backed enterprises.
Key players include Air Liquide, Linde, and Air Products and Chemicals, all of which have sustained investments in cryogenic distillation and isotope separation plants. Air Liquide continues to operate advanced cryogenic facilities for stable isotope production, with recent expansions to address medical and energy sector growth. Linde maintains a strong presence in Europe and Asia, leveraging expertise in large-scale cryogenic systems and partnering with research institutions for isotopic enrichment projects.
A notable strategic move in the past year includes the collaboration between Air Liquide and nuclear technology companies to secure supply chains for enriched isotopes used in next-generation reactor fuels and radiopharmaceuticals. Similarly, Air Products and Chemicals has announced upgrades to its cryogenic separation infrastructure in North America, enhancing capacity for rare gas and isotope extraction.
State-backed organizations play a critical role as well. In Russia, Rosatom continues to expand its isotope production and enrichment capabilities, exporting stable isotopes and collaborating internationally on supply security. China’s nuclear sector, through entities like China National Nuclear Corporation (CNNC), is rapidly scaling up cryogenic separation projects in line with national energy and healthcare priorities.
The sector is witnessing increasing joint ventures and research partnerships. For example, European consortia involving Linde are pursuing advancements in cryogenic isotope separation efficiency, targeting higher yields and energy reduction. There is also an uptick in public-private partnerships aimed at securing isotopic supply chains, especially for medical isotopes such as oxygen-18 and xenon-129.
Looking ahead, the next few years are expected to see intensified competition as demand surges for isotopes critical to fusion energy, quantum technology, and advanced diagnostics. The leading players are investing in process automation, digital monitoring, and modular facility design to ensure scalability and competitiveness. Global geopolitics, regulatory requirements, and technology licensing will further shape the landscape, but the incumbents’ deep expertise and capital base position them to maintain leadership in cryogenic isotopic separation.
Technological Innovations: Cryogenic Techniques and System Advancements
Cryogenic isotopic separation, a process that leverages ultra-low temperatures to exploit minute differences in physical properties among isotopes, is experiencing a wave of technological innovation as 2025 approaches. This technique is integral to sectors such as nuclear energy, medical isotope production, and emerging quantum technologies. The core principle relies on distillation or condensation at cryogenic temperatures, where volatility or phase-change differences allow for the efficient partitioning of isotopes such as deuterium from hydrogen, or oxygen-18 from natural oxygen.
Recent improvements in cryogenic engineering have focused on system efficiency, energy consumption, and scalability. Companies such as Air Liquide and Linde—global leaders in industrial gases and cryogenic technologies—are investing in modular, high-throughput distillation columns and advanced heat exchanger designs. These enable greater process control and throughput, essential for meeting the rising demand for isotopically enriched materials in both medical diagnostics and advanced materials manufacturing.
A notable trend in 2025 is the integration of digital process automation and real-time analytics. New-generation cryogenic plants deployed by Air Products and Praxair (now part of Linde) are equipped with advanced sensors and automated feedback systems. These allow for precise monitoring of pressure, temperature, and isotopic purity, resulting in improved yield and energy optimization. This digitalization is expected to reduce operating costs and carbon footprints, aligning with sustainability targets across the specialty chemicals and nuclear sectors.
Material science innovations are also reshaping cryogenic separation. The adoption of corrosion-resistant alloys and new insulation materials, championed by companies like Cryo Industries of America, is extending system lifespans and minimizing operational downtime. Enhanced cryogenic compressors and pumps, with improved reliability and lower maintenance requirements, are further supporting continuous, large-scale isotope production.
Looking ahead, the outlook for cryogenic isotopic separation is buoyed by its critical role in supplying key isotopes for fusion energy research, radiopharmaceuticals, and high-precision sensors. With ongoing investments in cryogenic infrastructure and process automation, major industry players are well-positioned to deliver incremental gains in efficiency and purity over the next several years. This technological momentum is expected to support expanded applications in both established and emerging markets worldwide.
Supply Chain Dynamics and Raw Material Considerations
Cryogenic isotopic separation is a cornerstone technology in the supply chain for enriched stable and radioactive isotopes, playing a pivotal role in sectors such as nuclear energy, medicine, and scientific research. The supply chain for cryogenic isotope separation systems in 2025 is characterized both by strategic bottlenecks and emerging opportunities, as global demand for isotopically enriched materials—especially uranium, deuterium, and specific noble gases—remains strong.
The raw materials essential for cryogenic isotopic separation are high-purity feedstocks of target elements—such as uranium hexafluoride for uranium enrichment, or methane and water for deuterium extraction. The procurement and transport of these feedstocks are tightly controlled by regulatory frameworks, especially for strategic or dual-use isotopes. Suppliers such as Orano and Urenco continue to dominate the upstream uranium supply, while companies like Air Liquide and Linde remain major providers of industrial gases and rare gas isotopes.
On the equipment side, the manufacture and maintenance of cryogenic distillation columns, compressors, and high-vacuum systems often depend on specialist suppliers for advanced alloys and precision control systems. These components are typically sourced from a select global network, which introduces vulnerabilities to geopolitical disruptions, particularly for high-technology alloys and electronic controls. In parallel, ongoing modernization efforts—such as automation and digital monitoring—are being implemented by industry leaders to improve yield and reliability, as indicated by public statements from Orano and Urenco.
Logistics and handling represent another critical node in the supply chain. The transport of isotopic feedstocks and separated products requires secure, certified containers and adherence to strict international protocols, especially when dealing with radioactive or hazardous substances. Firms like Orano and Urenco provide integrated logistics services to mitigate risks of contamination and diversion.
Looking ahead to the next few years, supply chain resilience will be tested by rising demand for enriched isotopes for medical diagnostics, fusion research, and advanced nuclear reactors. Strategic investments in cryogenic infrastructure and new purification technologies are anticipated, with a focus on reducing energy consumption and increasing throughput. Companies are expected to deepen partnerships with raw material suppliers and equipment manufacturers to secure long-term supply contracts and develop contingency plans. Such moves aim to ensure a stable pipeline of high-purity isotopes amid evolving regulatory and geopolitical landscapes.
Regulatory Environment and International Standards
The regulatory environment for cryogenic isotopic separation is shaped by stringent controls due to its critical role in nuclear energy, medical isotope production, and scientific research. As of 2025, oversight is largely guided by international non-proliferation agreements, safety standards, and national regulations that address the sensitive nature of isotope separation technologies, particularly those involving uranium enrichment and stable isotope production.
The International Atomic Energy Agency (IAEA) remains the principal body establishing global standards for the peaceful use of nuclear technologies, including cryogenic enrichment processes. The IAEA’s safeguards agreements require member states to declare and submit facilities using cryogenic isotopic separation to periodic inspections, with a focus on preventing diversion of nuclear materials for weapons purposes. In the past year, the IAEA has updated technical guidance for the application of safeguards to new and advanced enrichment facilities, reflecting the increased interest in high-throughput cryogenic systems.
On a regional level, the European Atomic Energy Community (Euratom) operates a parallel set of regulations for EU member states. Euratom’s framework mandates licensing, reporting, and real-time monitoring of facilities engaged in isotopic separation using cryogenic technologies, with harmonized safety and environmental standards across Europe. Compliance is enforced via regular audits and information exchange mechanisms within the EU.
In the United States, the U.S. Nuclear Regulatory Commission (NRC) regulates cryogenic isotope separation under 10 CFR Part 70 and Part 110, which govern the domestic use and export of nuclear materials and enrichment technology. The NRC, in coordination with the U.S. Department of Energy (DOE), has recently revised licensing requirements, emphasizing cybersecurity and supply chain transparency for advanced cryogenic systems, particularly those used in uranium enrichment and stable isotope production.
Globally, the Wassenaar Arrangement and the Nuclear Suppliers Group (NSG) maintain export control lists that include cryogenic isotopic separation equipment. These controls are expected to tighten in the coming years in response to technological advances, with member states proposing stricter end-user verification and traceability requirements.
Looking ahead, the convergence of international standards and increased digitalization of facility monitoring are set to enhance both the transparency and security of cryogenic isotopic separation operations. Stakeholders anticipate further harmonization of safety and non-proliferation frameworks, especially as global demand for medical and industrial isotopes expands. Collaborative efforts between regulatory bodies and technology providers will likely play a crucial role in shaping the regulatory landscape through 2025 and beyond.
Challenges: Technical, Economic, and Environmental Barriers
Cryogenic isotopic separation remains a cornerstone technology for the purification of stable and radioactive isotopes, with applications spanning nuclear power, medicine, and scientific research. However, as of 2025 and looking toward the next few years, the sector faces a confluence of technical, economic, and environmental barriers that challenge its broader adoption and optimization.
Technically, the cryogenic processes—most notably cryogenic distillation—require operation at extremely low temperatures, often below 77 K for nitrogen and even lower for hydrogen and its isotopes. Achieving and maintaining these conditions demands advanced cryogenic infrastructure and precise process control. The separation of isotopes with minimal mass differences, such as deuterium from hydrogen or uranium isotopes, necessitates tall columns and high reflux ratios, increasing both equipment size and complexity. Companies such as Air Liquide and Linde remain among the few with the expertise and infrastructure to design, construct, and operate these systems at industrial scale. Even so, the energy consumption for large-scale operations remains significant, and process efficiency is often hindered by the need to minimize isotope losses and contamination.
Economically, the capital and operational expenditures for cryogenic separation plants are substantial. High-purity distillation columns, specialized compressors, vacuum insulation, and safety systems drive up initial investment costs. Furthermore, the volatility of energy prices directly impacts operational costs, as cryogenic refrigeration is energy-intensive. The market for isotopically enriched materials is niche and cyclical, often tied to the fluctuating demands of nuclear power, radiopharmaceuticals, or national security priorities. Only a handful of producers globally, including ROSATOM, maintain the necessary scale for cost-effective production. Smaller entities often find it unfeasible to enter or expand in this market due to prohibitive costs and long return-on-investment timelines.
Environmental considerations are increasingly shaping the outlook for cryogenic isotopic separation. The process’s high energy requirements can result in a substantial carbon footprint, especially in regions where electricity is sourced from fossil fuels. Regulatory scrutiny concerning greenhouse gas emissions and operational safety is expected to intensify through the latter 2020s. Leading industrial gas suppliers, including Air Products, are investing in efficiency improvements and exploring integration with renewable energy sources to mitigate environmental impacts. However, retrofitting existing plants and developing next-generation low-carbon cryogenic technology remains a slow and capital-intensive endeavor.
In summary, overcoming the intertwined technical, economic, and environmental barriers will be essential for the sustained viability and expansion of cryogenic isotopic separation. Collaboration among technology providers, end-users, and regulators will likely define progress in the coming years.
Investment and Partnership Opportunities in 2025 and Beyond
The cryogenic isotopic separation sector is experiencing a pivotal period of investment and partnership activity as global demand for high-purity isotopes intensifies across energy, healthcare, and scientific research domains. In 2025 and the following years, the market outlook is shaped by both governmental initiatives and private enterprise strategies aimed at scaling up advanced separation technologies, enhancing process efficiencies, and addressing supply chain security.
Strategic investments are increasingly concentrated on expanding cryogenic infrastructure, particularly with the rise of nuclear energy projects and the push for stable isotope production for medical diagnostics, radiotherapy, and quantum computing applications. Leading organizations such as Orano and ROSATOM are actively modernizing existing facilities and exploring joint ventures with technology innovators to accelerate deployment of next-generation cryogenic separation columns. In parallel, national laboratories and state-backed entities are fostering partnerships with industrial players to commercialize new isotopic enrichment processes and ensure resilient supply chains.
For example, Orano continues to invest in cryogenic separation capabilities within its Tricastin complex, supporting both nuclear fuel cycle needs and the growing market for stable isotopes. Meanwhile, ROSATOM has announced collaborative projects with international partners to develop advanced cryogenic techniques for isotopic enrichment, particularly relevant for emerging fusion energy initiatives and the global push for carbon-neutral technologies. In North America, entities leveraging cryogenic expertise are seeking cross-sector alliances with medical isotope distributors and healthcare providers to secure long-term supply agreements.
Private capital is also flowing into start-ups and scale-ups that offer modular and energy-efficient cryogenic separation units, promising greater flexibility and reduced operational costs. These companies are targeting both established sectors (such as nuclear medicine and industrial gas production) and new frontiers, including quantum technologies that require ultra-high-purity isotopes. The competitive landscape is further energized by government grant programs and international consortia, which are fostering knowledge exchange and risk-sharing in both research and commercialization stages.
Looking ahead, successful investment and partnership models are likely to be those that integrate upstream supply reliability with downstream application expertise, while prioritizing sustainability and regulatory compliance. Stakeholders who align with major industry leaders, leverage public-private frameworks, and invest in scalable cryogenic technologies will be well-positioned to capture value as demand for isotopic separation accelerates through 2025 and beyond.
Future Outlook: Disruptive Trends and Long-Term Market Potential
Cryogenic isotopic separation, a cornerstone technology for producing high-purity isotopes essential in nuclear energy, medicine, and scientific research, is poised for transformative developments in 2025 and the years immediately ahead. As the demand for stable and radioactive isotopes intensifies—driven by nuclear reactor fuel requirements, medical diagnostics, and quantum computing materials—industry stakeholders are accelerating innovation to expand capacity and boost process efficiency.
A key disruptive trend involves the integration of advanced automation and digital monitoring into cryogenic distillation plants. Leading suppliers such as Linde and Air Liquide are investing in process control systems that leverage real-time data analytics and machine learning to optimize separation yields, reduce operational costs, and increase plant flexibility. This digitalization is expected to facilitate rapid response to shifting isotope specifications, particularly as new nuclear reactor designs and radiopharmaceuticals enter the market.
Another major development is the push for energy-efficient operations. Traditional cryogenic separation remains energy-intensive, but ongoing R&D seeks to minimize environmental footprint. Companies such as Linde are exploring novel heat integration techniques and advanced refrigeration cycles to reduce power consumption per unit of separated isotope. This sustainability imperative aligns with global decarbonization goals and is anticipated to become a differentiator for suppliers vying for contracts with governments and large research consortia.
The global geopolitical landscape is also shaping the sector’s outlook. Supply chain disruptions and export controls on strategic isotopes are prompting investments in domestic isotopic separation capabilities, particularly in the United States, Europe, and East Asia. Several national laboratories and public-private partnerships are scaling up cryogenic separation infrastructure to ensure a robust, sovereign supply of critical isotopes such as deuterium, enriched xenon, and isotopically pure gases for quantum technologies.
Looking forward, the market potential for cryogenic isotopic separation is expected to expand as applications diversify. Emerging fields like quantum computing, where isotopically pure silicon and germanium are crucial, are opening new high-value markets. Similarly, the growth of next-generation nuclear reactors—including small modular reactors (SMRs)—is anticipated to drive demand for isotopically tailored fuels. Industry bodies such as the World Nuclear Association predict that technological advancements and policy-driven capacity expansions will shape a robust, innovation-driven market over the next five years, with cryogenic processes remaining at the forefront of isotopic separation solutions.