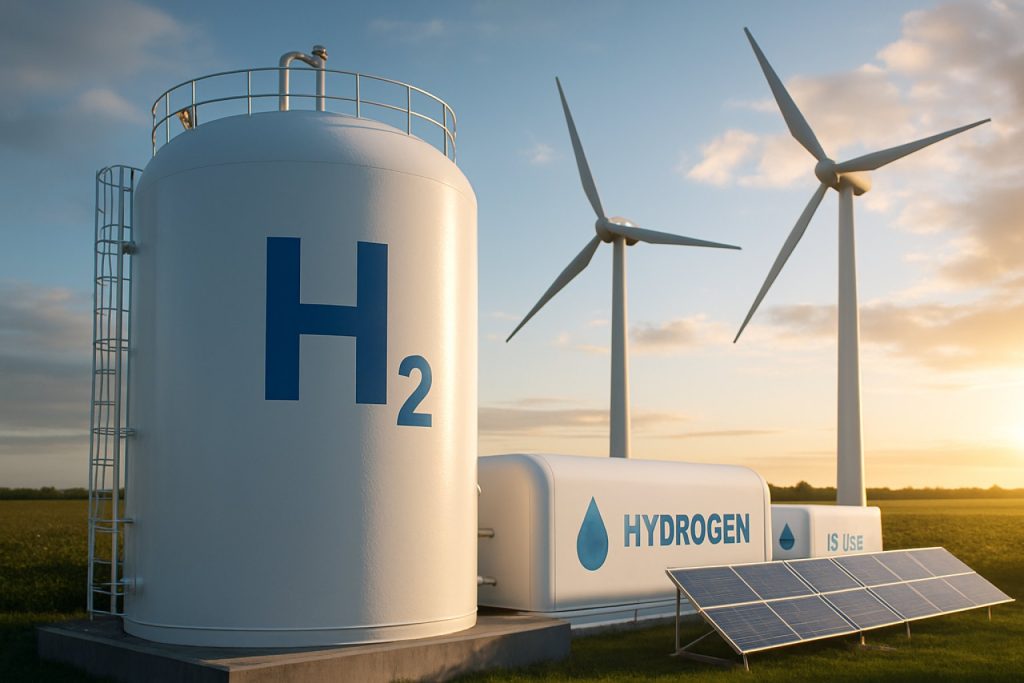
Table of Contents
- Executive Summary: 2025 Snapshot and Industry Trajectory
- Market Size, Growth Forecasts, and Regional Hotspots (2025–2030)
- Latest Technological Advances in Synthetic Biohydrogen Production
- Key Players and Strategic Partnerships: Who’s Leading the Charge?
- Regulatory Landscape and Global Sustainability Initiatives
- Novel Feedstocks and Process Optimization Techniques
- Challenges: Economic, Technical, and Scalability Barriers
- Emerging Applications Across Industries
- Investment Trends and Funding Landscape
- Future Outlook: Disruptive Innovations and Long-Term Market Scenarios
- Sources & References
Executive Summary: 2025 Snapshot and Industry Trajectory
Synthetic biohydrogen production systems are poised for a transformative role in the global clean energy landscape in 2025 and beyond. As demand for sustainable hydrogen accelerates, industry players and research institutions are advancing integrated biological and synthetic processes to scale up biohydrogen output. Unlike conventional hydrogen generation—which is still heavily reliant on fossil fuels—synthetic biohydrogen leverages engineered microorganisms, optimized bioreactors, and sometimes hybrid approaches that combine biochemical and electrochemical pathways to convert renewable feedstocks into molecular hydrogen.
In 2025, key milestones focus on pilot- and demonstration-scale facilities. Several companies are actively moving from laboratory validation to commercial feasibility. For instance, Siemens is collaborating with partners to develop bioreactor systems that integrate renewable electricity and engineered microbial platforms for efficient hydrogen production, seeking to reduce both carbon intensity and operating costs. Meanwhile, Air Liquide is investing in R&D partnerships targeting enzymatic and microbial solutions for decentralized hydrogen generation, with pilot units expected to reach operational status by late 2025.
The European Union’s significant funding initiatives are catalyzing deployment, with the Clean Hydrogen Partnership supporting multiple consortia focused on synthetic biology routes for hydrogen generation. In Asia, Toyota Motor Corporation has announced collaborative research aimed at integrating synthetic biohydrogen into their wider hydrogen ecosystem, assessing both cost reduction and scalability for the transport sector.
Technical advances in 2025 include genetic optimization of hydrogenase enzymes, improved metabolic pathways for higher hydrogen yields, and the development of robust, modular bioreactors. These innovations are expected to push system efficiencies to new benchmarks—potentially reaching conversion efficiencies above 60%—and to broaden the feedstock base to include agricultural residues and industrial byproducts.
Looking ahead to the next several years, the sector is expected to progress towards commercial-scale deployment. Industry forecasts anticipate the first commercial synthetic biohydrogen plants to be operational by 2027–2028, with early adopters likely to be in regions with strong policy support and access to abundant biomass or renewable electricity. Key challenges remain, notably in process scale-up, feedstock logistics, and economic competitiveness relative to electrolysis and fossil-based hydrogen. Nonetheless, strategic alliances between technology developers, utilities, and end-users are expected to accelerate market readiness and drive down costs.
Overall, 2025 marks a pivotal year for synthetic biohydrogen production systems, setting the stage for broader industrial adoption and forming an integral component of the emerging green hydrogen economy.
Market Size, Growth Forecasts, and Regional Hotspots (2025–2030)
The global market for synthetic biohydrogen production systems is anticipated to experience robust growth between 2025 and 2030, propelled by escalating demand for clean hydrogen, government decarbonization policies, and rapid technological advances in bioprocessing platforms. In 2025, biohydrogen remains a small but rapidly expanding segment within the broader hydrogen market, with pilot and demonstration plants emerging in key regions. The market is projected to transition from pilot to early commercial scale by 2030, reaching cumulative installed capacities in the low hundreds of megawatts globally.
Growth is driven by the integration of advanced synthetic biology, metabolic engineering, and fermentation technologies to engineer microorganisms—such as cyanobacteria, algae, and bacteria—for efficient, scalable hydrogen production. Europe and Asia-Pacific are leading regional hotspots due to significant public and private investments. The European Union’s focus on sustainable hydrogen and the funding mechanisms under its Green Deal have accelerated projects involving synthetic and bio-based hydrogen. Companies such as Novozymes are advancing enzymatic and microbial solutions, with collaborations targeting improved conversion efficiencies and process economics.
In Asia, Japan and South Korea are actively supporting R&D initiatives and demonstration-scale facilities, aiming to establish leadership in hydrogen import/export and supply chains. For example, Japan’s “Green Growth Strategy” includes substantial funding for biohydrogen pilot projects and partnerships with biotechnology firms. China, meanwhile, is scaling up biohydrogen research as part of its broader hydrogen roadmap, integrating domestic technology providers and academic institutions.
North America is also emerging as a key market, supported by U.S. Department of Energy initiatives and a surge in venture-backed startups focusing on synthetic biology-driven hydrogen production. Companies such as Genomatica and LanzaTech are developing microbial and gas fermentation platforms that could enable cost-competitive biohydrogen by the late 2020s. The U.S. Inflation Reduction Act and related state-level incentives are expected to further catalyze investment and project deployment.
From 2025 onward, market growth will depend on successful scale-up from laboratory and pilot to commercial demonstration; improvements in yield, process stability, and feedstock flexibility; and competitive hydrogen production costs relative to electrolysis and fossil-based alternatives. With several regional clusters, strong policy support, and visible industry participation, synthetic biohydrogen production systems are poised for significant expansion, particularly in Europe, East Asia, and North America, through 2030.
Latest Technological Advances in Synthetic Biohydrogen Production
The landscape of synthetic biohydrogen production systems is undergoing rapid evolution as research and industry intensify efforts to decarbonize energy and chemical processes. In 2025, several breakthroughs are shaping the development and deployment of these technologies, with a focus on scalability, efficiency, and integration with renewable resources.
Recent advances in genetic engineering have enabled the construction of microbial platforms with enhanced hydrogen yields. Notably, engineered strains of Escherichia coli and cyanobacteria are being used to convert biomass-derived sugars or even CO2 directly into hydrogen with improved efficiency. For example, companies such as LanzaTech are leveraging synthetic biology to develop gas fermentation systems where engineered microbes produce hydrogen and other chemicals from industrial off-gases, effectively closing the carbon loop and reducing emissions.
Another significant trend is the adoption of photobiological systems—where genetically modified algae or cyanobacteria generate hydrogen using sunlight and water. This approach has gained traction due to its potential for direct solar-to-hydrogen conversion without intermediate electricity generation. Novozymes, a leader in industrial biotechnology, is collaborating with partners to optimize enzyme pathways and cell chassis for higher hydrogen productivity, aiming for commercial-scale demonstration by 2026.
Electro-microbial hybrid systems are also emerging, combining the robustness of electrolysis with the selectivity of engineered microbes. These systems channel renewable electricity to drive microbial metabolic pathways, converting simple feedstocks like CO2 and water into hydrogen. Industrial biotechnology firms such as DuPont are investing in pilot-scale facilities to validate the economic and environmental benefits of such hybrid processes, with results expected to inform scaling decisions in the next two to three years.
Integration with circular economy principles is a major focus: synthetic biohydrogen systems are increasingly designed to utilize waste streams—such as agricultural residues, municipal solid waste, or captured CO2—as feedstocks, reducing both operating costs and environmental impact. This aligns with global industry initiatives and regulatory incentives advancing in 2025 to promote low-carbon hydrogen.
Looking forward, the outlook for synthetic biohydrogen systems is positive, with demonstration plants planned across North America, Europe, and Asia. Industry bodies such as Hydrogen Europe anticipate that by the late 2020s, these systems could reach commercialization, contributing significantly to the global hydrogen supply and supporting broader decarbonization goals.
Key Players and Strategic Partnerships: Who’s Leading the Charge?
The synthetic biohydrogen production landscape in 2025 is characterized by a convergence of biotechnology, energy, and engineering firms forming strategic alliances to accelerate commercialization. As nations prioritize low-carbon hydrogen, several key players have emerged at the forefront, leveraging genetic engineering, advanced bioreactors, and integrated systems for scalable biohydrogen generation.
Among global leaders, Shell stands out with its investments in microbial hydrogen production and partnerships with synthetic biology startups. The company’s recent collaborations focus on upscaling processes using engineered cyanobacteria and algae, aiming to integrate biohydrogen into existing hydrogen supply chains. Similarly, Air Liquide has intensified its activities, forming joint ventures with academic spin-offs to optimize photobiological and dark fermentation routes for industrial-scale hydrogen production.
In the US, ExxonMobil has announced pilot programs using engineered microbes for biohydrogen synthesis, targeting both blue and green hydrogen markets. Their R&D alliances with university consortia emphasize metabolic pathway engineering for higher hydrogen yields and reduced operational costs. On the European front, BASF is channeling resources into synthetic biology platforms, seeking to commercialize enzyme-driven hydrogen generation and to develop robust microbial strains for continuous production.
Strategic partnerships are also defining the sector’s trajectory. For instance, TotalEnergies has joined forces with bioengineering firms to co-develop pilot plants, blending synthetic biohydrogen with renewable hydrogen infrastructure. These collaborative ventures often involve regional governments and public research institutes, reflecting a broader push towards open innovation ecosystems. In Asia, Toyota Motor Corporation is exploring biohydrogen as part of its decarbonization roadmap, working with local biotech companies to integrate biohydrogen into fuel cell vehicle supply chains.
Looking ahead, these alliances are expected to intensify as synthetic biohydrogen systems move from demonstration to early commercial deployment. Regulatory incentives, such as the EU’s Renewable Energy Directive and US federal funding for clean hydrogen hubs, are catalyzing further investment and cross-sector partnerships. The outlook for 2025 and the subsequent years points to rapid scaling, with leading players positioning themselves through intellectual property, supply agreements, and technology licensing to capture emerging market opportunities in the global hydrogen economy.
Regulatory Landscape and Global Sustainability Initiatives
The regulatory landscape for synthetic biohydrogen production is rapidly evolving as nations and industry stakeholders align with ambitious decarbonization targets for 2025 and beyond. Biohydrogen—produced via engineered microorganisms or enzymatic processes using renewable biomass or waste substrates—has attracted policy attention for its potential to deliver carbon-neutral or even carbon-negative hydrogen. As of 2025, regulatory frameworks are converging on criteria that prioritize lifecycle greenhouse gas (GHG) reduction, feedstock sustainability, and traceability of renewable inputs.
The International Energy Agency highlights biohydrogen as a key component in global hydrogen roadmaps, with updated guidelines emphasizing certification schemes for low-carbon hydrogen, including synthetic biohydrogen. The European Union has advanced its regulatory approach through the Renewable Energy Directive (RED II and successor RED III) and the Hydrogen Strategy, which set mandatory sustainability and GHG reduction thresholds for bio-based hydrogen. These directives are influencing uptake of third-party certification and mass-balance tracking for biohydrogen producers, ensuring compliance with rigorous environmental and social standards.
In 2025, the European Clean Hydrogen Alliance and the Clean Hydrogen Partnership continue to coordinate public-private initiatives and funding, supporting pilot and demonstration plants that use engineered microbial systems for hydrogen production. These projects are designed to meet evolving EU Taxonomy criteria for sustainable investment, further linking regulatory compliance with access to capital. National governments such as Germany and the Netherlands are implementing subsidy schemes and procurement preferences for certified renewable hydrogen, including synthetic biohydrogen, under their respective hydrogen strategies and climate action plans.
Outside Europe, the U.S. Department of Energy is piloting the Clean Hydrogen Production Standard (CHPS), which sets specific carbon intensity benchmarks for hydrogen pathways, explicitly incorporating biohydrogen routes. The CHPS is expected to influence eligibility for federal funding and tax credits, such as those in the Inflation Reduction Act. In Asia, Japan’s Green Growth Strategy and South Korea’s Hydrogen Economy Roadmap both recognize biohydrogen as a qualifying pathway for clean hydrogen targets, with regulatory frameworks evolving to define sustainability criteria and incentivize R&D investment.
Globally, sustainability initiatives are increasingly aligned with United Nations Sustainable Development Goals (SDGs), particularly SDG 7 (Affordable and Clean Energy) and SDG 13 (Climate Action). Industry bodies such as the International Association for Hydrogen Energy are collaborating with regulators to develop standardized certification and monitoring protocols for synthetic biohydrogen. Looking forward, the next few years will see growing harmonization of definitions, certification standards, and data reporting requirements, unlocking international trade and investment in sustainable biohydrogen systems. For technology developers, compliance with these evolving frameworks is becoming a prerequisite for market access and scale-up in the emerging global hydrogen economy.
Novel Feedstocks and Process Optimization Techniques
In 2025, the landscape for synthetic biohydrogen production systems is marked by rapid advancements in both novel feedstock utilization and process optimization, driven by pressing global decarbonization targets and growing commercial interest in green hydrogen. Synthetic biohydrogen production leverages engineered microorganisms or enzymatic systems to convert renewable biomass or waste streams into molecular hydrogen, offering a sustainable alternative to traditional fossil-fuel-derived hydrogen.
One of the most significant trends is the expansion of feedstock diversity beyond conventional agricultural residues. Companies and research consortia are intensifying efforts to valorize municipal solid waste, food industry by-products, and even industrial CO2 emissions as viable carbon sources for biohydrogen production. For example, Shell is actively collaborating with bioengineering firms to assess the feasibility of integrating waste-to-hydrogen platforms within existing biorefinery infrastructures. Additionally, BASF and partners are advancing the use of lignocellulosic residues and specifically engineered algae strains to boost hydrogen yields, addressing both feedstock sustainability and process scalability.
Process optimization is concurrently witnessing major breakthroughs, particularly in metabolic engineering and bioreactor design. Cutting-edge genetic modification of microbes—such as Clostridium and cyanobacteria—is enabling higher substrate-to-hydrogen conversion efficiencies by minimizing competing metabolic pathways. This is complemented by the deployment of next-generation continuous-flow bioreactors equipped with real-time monitoring and automated control systems, which are being piloted by organizations like Air Liquide to enhance process reliability and reduce operational costs.
Another frontier is the integration of synthetic biology with electrochemical and photobiological methods, facilitating hybrid systems that harness both biological and renewable electrical inputs to maximize hydrogen output. Novozymes is pioneering enzyme-catalyzed hydrogen production modules that can be embedded in modular units, aiming for deployment in distributed energy networks by 2026. These modularized systems are expected to accelerate adoption in decentralized settings, including remote industrial sites and off-grid communities.
Looking ahead to the next few years, the outlook for synthetic biohydrogen production systems is highly promising. The convergence of advanced feedstocks, process intensification, and digitalization is forecasted to drive down the levelized cost of biohydrogen, positioning it as a competitive player within the broader hydrogen economy. With the European Union’s ongoing funding and demonstration programs, and major industrial stakeholders increasing investment, commercialization of scalable, synthetic biohydrogen solutions is anticipated to gain significant momentum by the late 2020s.
Challenges: Economic, Technical, and Scalability Barriers
Synthetic biohydrogen production systems, which leverage genetically engineered microorganisms or enzymatic processes for hydrogen generation, are gaining momentum as a core pathway for sustainable energy. However, as of 2025, the sector faces significant economic, technical, and scalability barriers that could limit near-term deployment and impact.
Economically, the most pronounced challenge lies in the high cost of bioreactor infrastructure and feedstock. Advanced fermentation and photobioreactor systems, required for optimal yields, involve substantial upfront capital expenditure and ongoing operational costs. Commercial leaders such as Novozymes and DSM, both active in industrial biotechnology, have acknowledged that economies of scale are yet to be achieved for most biohydrogen platforms. Furthermore, the synthetic biology required to engineer efficient hydrogen-producing strains remains resource-intensive, with expensive gene editing, screening, and validation processes.
- Technical Barriers: The engineering of robust microorganisms capable of withstanding industrial conditions, while maintaining high hydrogen productivity, is a central technical challenge. Genetic instability, sensitivity to contaminants, and low tolerance to end-product inhibition often limit the longevity and efficiency of production strains. As reported by various industry players, including Novozymes, optimizing metabolic pathways for hydrogen output without sacrificing cell viability or growth rates remains a complex task.
- Process Integration and Scale-Up: Transitioning from laboratory-scale demonstrations to pilot and commercial-scale systems introduces further issues. Maintaining consistent yields at scale, managing mass transfer (especially for gaseous hydrogen), and preventing contamination in large bioreactors require significant engineering advances. Companies are investing in process intensification and modular bioreactor designs, but as of 2025, most demonstrations remain below the 10,000-liter scale.
- Feedstock Competition and Sustainability: The reliance on organic substrates (such as sugars or agricultural waste) for microbial hydrogen production introduces feedstock sourcing challenges. Competing demand from other bio-based industries and variable feedstock quality can impact both costs and process reliability, as noted by industrial biotechnology organizations such as DSM.
Looking ahead to the next few years, ongoing R&D efforts focus on strain development, process automation, and integration of renewable feedstocks. However, unless breakthroughs in cost reduction and process robustness are achieved, synthetic biohydrogen production systems are likely to remain limited to pilot and demonstration projects through the mid-2020s. The sector’s progress will hinge on collaborative industry efforts and supportive policy frameworks to overcome these persistent barriers.
Emerging Applications Across Industries
Synthetic biohydrogen production systems are poised to play a transformative role across diverse industries as the global push toward decarbonization accelerates in 2025 and the subsequent years. These systems, which leverage engineered microorganisms or enzymatic processes to generate hydrogen from renewable or waste biomass, are drawing interest from sectors seeking sustainable alternatives to fossil-derived hydrogen. Notably, the energy, transportation, chemical manufacturing, and waste management industries are among the early adopters pursuing pilot projects and commercial-scale initiatives.
In the energy sector, biohydrogen is being integrated into green hydrogen portfolios to support grid balancing and renewable energy storage. Companies such as Shell and Air Liquide have announced investments in R&D partnerships focused on microbial and enzymatic hydrogen production technologies, aiming to supplement electrolytic hydrogen with cost-effective bio-based alternatives. These initiatives are expected to help meet tightening regulatory requirements on carbon emissions and facilitate the scaling of hydrogen infrastructure.
The transportation industry is exploring biohydrogen as a feedstock for fuel cell vehicles, particularly in heavy-duty and maritime segments where energy density and rapid refueling are critical. Toyota Motor Corporation is collaborating with synthetic biology firms to evaluate the integration of biohydrogen into its hydrogen-powered vehicle platforms, with pilot trials anticipated to expand in 2025. Similarly, several European ports are working with technology providers to trial biohydrogen-powered harbor equipment and vessels as part of broader decarbonization strategies.
Within the chemicals sector, biohydrogen offers a route to low-emission ammonia, methanol, and specialty chemicals production. BASF and Nestlé are participating in consortia aiming to demonstrate the viability of synthetic biohydrogen as a process feedstock, with initial commercial-scale demonstration units expected by 2026. This could reduce reliance on steam methane reforming and significantly lower the carbon footprint of key industrial products.
Another emerging application is in waste valorization, where biohydrogen systems are deployed at municipal waste treatment and agricultural sites to convert organic residues into hydrogen. Veolia is testing integrated waste-to-hydrogen modules, aligning with circular economy objectives and offering new revenue streams for waste operators.
Looking ahead, the outlook for synthetic biohydrogen production systems is shaped by rapid advances in synthetic biology, supportive policy frameworks, and growing industry demand for renewable hydrogen. Demonstration projects in 2025 and beyond are expected to validate techno-economic feasibility, paving the way for broader adoption across sectors as part of the global transition to clean energy and materials.
Investment Trends and Funding Landscape
Investment in synthetic biohydrogen production systems is intensifying as global interest in low-carbon hydrogen solutions accelerates into 2025. Major public and private funding streams are converging to support both startup innovation and scale-up of demonstration projects, particularly where synthetic biology and renewable feedstocks intersect.
In the past year, several large-scale initiatives have been announced, including multimillion-dollar grants and joint ventures. For example, the Shell GameChanger program has identified biohydrogen as a strategic priority, seeking partnerships with synthetic biology startups to develop scalable, low-emission hydrogen production technologies. Similarly, TotalEnergies has stated its intent to increase investments in advanced hydrogen, specifically highlighting synthetic routes and bio-based feedstocks in its 2025 outlook.
On the venture capital front, funding for synthetic biohydrogen startups is growing steadily. 2024 saw several Series A and B rounds exceeding $30 million in the US and EU, with investors targeting technologies that utilize engineered microorganisms or enzymatic pathways to convert biomass or waste into hydrogen. Notably, Novozymes, a leader in industrial biotechnology, has expanded its corporate venture arm’s focus to include companies developing synthetic biology platforms for hydrogen production. This trend is expected to accelerate in 2025 as the hydrogen market matures and synthetic biological routes move from proof-of-concept to pilot scale.
Public sector funding is also robust. The European Union’s Clean Hydrogen Partnership, a joint initiative between industry and the European Commission, has earmarked over €1 billion for innovative hydrogen projects through 2027, with a significant allocation towards biological and synthetic hydrogen pathways (Clean Hydrogen Partnership). Meanwhile, the US Department of Energy continues to support synthetic biohydrogen R&D under its Hydrogen Shot initiative, offering grants and technical assistance for projects demonstrating cost-effective, scalable processes (U.S. Department of Energy).
Looking ahead, the funding landscape for synthetic biohydrogen appears increasingly competitive and well-resourced. Corporate commitments, venture investments, and government programs are expected to converge, driving the first commercial-scale deployments before 2030. The next few years will be crucial for technology validation and cost reduction, with investors closely watching for breakthroughs in efficiency, feedstock flexibility, and integration with renewable energy systems.
Future Outlook: Disruptive Innovations and Long-Term Market Scenarios
Synthetic biohydrogen production systems are emerging as a disruptive force in the hydrogen economy, with innovations in microbial engineering, bioreactor design, and process integration poised to reshape the industry landscape by and beyond 2025. Unlike conventional hydrogen production, which is largely dependent on fossil fuel-based steam methane reforming, synthetic biohydrogen leverages genetically engineered microorganisms or consortia to convert renewable feedstocks—such as agricultural residues, industrial waste, or even carbon dioxide—directly into hydrogen.
Recent advances in synthetic biology and metabolic engineering have enabled the creation of microbial strains with optimized hydrogenase pathways, higher substrate utilization efficiencies, and enhanced tolerance to industrial-scale conditions. Companies and research consortia are increasingly focusing on scalable photobiological and dark fermentation systems, with several pilot and demonstration projects underway in Asia, North America, and Europe. For example, industry bodies like the International Energy Agency (IEA) have identified synthetic biohydrogen as a key technology pathway in their Hydrogen Roadmap, highlighting its potential to deliver carbon-negative hydrogen when coupled with carbon capture and utilization or sequestration.
In 2025, synthetic biohydrogen systems are expected to reach critical technical milestones, such as continuous operation in modular bioreactors, improved conversion yields (targeting >5 mol H2/mol substrate for certain feedstocks), and integration with renewable energy sources for process intensification. Several commercial players, such as Shell, are actively exploring biohydrogen partnerships, while global energy and chemical companies are investing in R&D collaborations to de-risk scale-up challenges. Key market drivers include the need to decarbonize hard-to-abate sectors (e.g., steelmaking, chemicals), growing policy support for green hydrogen, and the potential for distributed, on-site hydrogen generation in rural or off-grid locations.
- Increased investment in pilot-scale bioreactors and demonstration plants is anticipated through 2025-2027, with focus on process reliability and lifecycle emissions assessment.
- Advancements in microbial consortia engineering may enable the use of mixed waste streams, further reducing feedstock costs and expanding deployment scenarios.
- Integration with existing biogas or biomass plants is expected to accelerate, leveraging infrastructure and supply chain synergies.
Looking toward the next few years, the outlook for synthetic biohydrogen production systems is strongly positive. As technical bottlenecks are addressed and regulatory frameworks evolve to recognize biohydrogen’s unique attributes, commercialization is expected to accelerate. Strategic alliances between technology developers, utilities, and end users will be crucial in bringing these disruptive systems to market, positioning synthetic biohydrogen as a cornerstone of the low-carbon hydrogen landscape for the late 2020s and beyond.