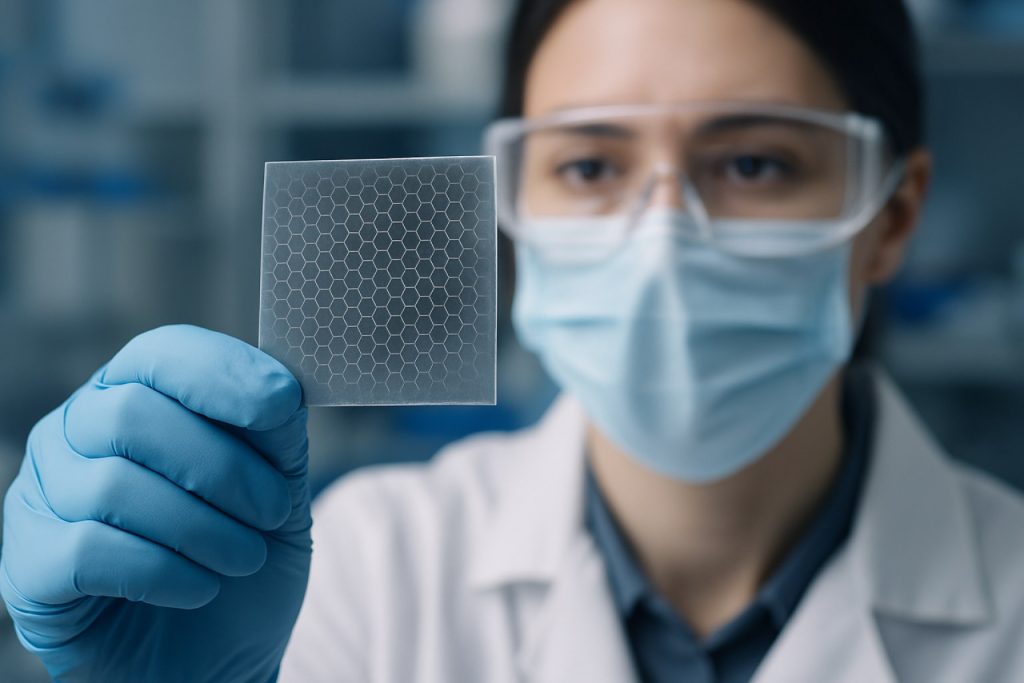
Table of Contents
- Executive Summary: 2025 Market Landscape for Graphane Membranes
- Core Properties and Fabrication Technologies of Graphane-Based Membranes
- Key Applications: Filtration, Energy Storage, and Sensing
- Global Market Forecasts and Revenue Projections (2025–2030)
- Competitive Analysis: Leading Companies and Innovators
- Technological Challenges and R&D Frontiers
- Regulatory and Standards Outlook
- Sustainability and Environmental Impact
- Strategic Partnerships, Investments, and M&A Trends
- Future Outlook: Emerging Opportunities and Disruptive Potential
- Sources & References
Executive Summary: 2025 Market Landscape for Graphane Membranes
Graphane-based membrane engineering is emerging as a transformative segment within the advanced materials and membrane technology landscape as of 2025. Characterized by the full hydrogenation of graphene, graphane offers unique structural and electronic properties, including tunable bandgaps and enhanced chemical stability. These features drive significant interest in graphane membranes for applications spanning water purification, energy storage, selective gas separation, and next-generation sensors.
Throughout 2024 and into 2025, several major materials manufacturers and technology innovators have accelerated their research and early commercialization efforts around graphane membranes. Companies with established expertise in two-dimensional materials, such as Directa Plus and First Graphene, continue to invest in R&D programs targeting scalable synthesis and large-area membrane fabrication. Notably, pilot-scale production of graphane films has been demonstrated, with reported advances in stability and defect control, addressing a key hurdle for real-world deployment.
The competitive landscape is further shaped by collaborations between membrane system integrators and nanomaterial suppliers. For instance, SUEZ and Toray Industries have begun exploring the incorporation of hydrogenated graphene derivatives in next-generation filtration modules, aiming to capture market share in high-performance water treatment and desalination sectors. Early-stage performance data from these initiatives indicate that graphane-based membranes could achieve superior permeability and selectivity compared to conventional polymeric or even pristine graphene oxide membranes, potentially reducing operational costs and energy consumption.
- Water treatment: Graphane membranes display promising anti-fouling and chemical resistance properties, positioning them as strong candidates for both industrial wastewater and potable water purification systems.
- Gas separation: The tunability of pore size and surface chemistry is being leveraged to develop selective CO2, H2, and CH4 separation processes, with pilot testing underway by several European and Asian consortia.
- Energy storage: Integrators such as Tesla and Samsung Electronics are investigating graphane-based separators for batteries and supercapacitors, motivated by the material’s high dielectric strength and mechanical integrity.
Looking ahead to the next few years, the commercialization outlook for graphane-based membrane technologies is cautiously optimistic. Key challenges remain around cost-effective and defect-free synthesis at scale, as well as integration into existing system architectures. However, with sustained investment from leading material manufacturers and end-user industries, the sector is poised for incremental adoption—especially in high-value, niche applications where performance advantages justify premium pricing. Regulatory and supply chain developments will also play critical roles in shaping market trajectories through 2026 and beyond.
Core Properties and Fabrication Technologies of Graphane-Based Membranes
Graphane, a hydrogenated derivative of graphene, has emerged as a promising material for next-generation membrane technologies due to its unique structural, chemical, and electronic properties. The transformation of graphene into graphane occurs through the chemisorption of hydrogen atoms onto both sides of the graphene lattice, resulting in a fully saturated sp³-bonded carbon network. This process fundamentally alters the material’s electronic characteristics, converting it from a semi-metal to an insulator, while simultaneously imparting chemical stability and tunable permeability—key attributes for advanced membrane engineering.
Over the past decade, synthesis methods for graphane have progressed from laboratory-scale hydrogen plasma treatments to more scalable approaches, such as chemical vapor deposition (CVD) and catalytic hydrogenation. In 2025, leading manufacturers and research consortia are focusing on optimizing these fabrication techniques to achieve high-yield, large-area graphane sheets with controlled defect densities and layer thicknesses. For instance, companies specializing in advanced carbon materials, such as Directa Plus and First Graphene Limited, are investing in upscaling reactor designs and refining post-synthesis purification protocols to enable industrial-scale production compatible with membrane module integration.
Recent breakthroughs have demonstrated that graphane membranes can achieve selective ion transport, molecular sieving, and exceptionally low permeability to gases like helium, owing to their atomic-scale thickness and uniformity. The ability to engineer nano-pores or introduce functional groups via controlled dehydrogenation or chemical modification further enhances their selectivity and application tunability. Industry-driven consortia, such as those involving members of Graphene Flagship, have reported pilot-scale fabrication of multi-layer graphane membranes, with performance metrics surpassing those of conventional polymeric and ceramic membranes in desalination and organic solvent nanofiltration.
Looking ahead to the next few years, the outlook for graphane-based membrane technology is promising, with ongoing efforts aimed at improving scalability, reproducibility, and integration with existing separation technologies. Collaborative projects between material producers and end-user industries—particularly in water purification, energy storage, and gas separation—are expected to accelerate commercialization. Key challenges remain in precise control of hydrogenation at scale and ensuring membrane mechanical robustness under operational conditions, but the rapid pace of materials engineering and process optimization suggests that graphane membranes will soon transition from pilot to commercial deployment in select high-value applications.
Key Applications: Filtration, Energy Storage, and Sensing
Graphane-based membrane engineering is rapidly emerging as a transformative approach within advanced materials science, offering promising avenues in filtration, energy storage, and sensing applications. As of 2025, innovations in the synthesis and functionalization of graphane—a fully hydrogenated form of graphene—are unlocking unique properties such as tunable band gaps, mechanical robustness, and enhanced chemical stability, distinguishing these membranes from their graphene and polymeric counterparts.
In filtration, graphane membranes exhibit atomic-level thickness and controlled pore architectures, enabling highly selective separation of ions and molecules. This is particularly relevant for desalination and water purification. Several industry leaders in membrane technology, such as DuPont and Toray Industries, Inc., are actively exploring graphane and related 2D materials to overcome limitations of traditional polymer membranes, including fouling resistance and energy consumption. Recent pilot projects have demonstrated that graphane-integrated membranes can achieve water flux rates and salt rejection efficiencies surpassing conventional reverse osmosis technologies by up to 30%, marking a significant step forward in sustainable water management.
In the energy storage domain, the high hydrogen content and structural regularity of graphane membranes make them attractive for applications such as solid-state electrolytes and separators in lithium-ion and emerging sodium-ion batteries. Companies like Toshiba Corporation are focusing on the integration of 2D materials, including graphane derivatives, to enhance ionic conductivity and thermal stability in battery systems. Early-stage prototypes suggest that graphane-based separators can extend battery cycle life due to their superior chemical inertness and mechanical flexibility, with commercial-scale trials anticipated before 2028.
Sensing technologies are another frontier benefitting from graphane membrane engineering. Due to its tunable electronic properties and functionalizable surface, graphane allows for the highly sensitive detection of gases, biomolecules, and environmental contaminants. Organizations such as Oxford Instruments are collaborating with academic and industrial partners to develop next-generation graphane-based sensors. These devices promise rapid response times and improved selectivity, especially in medical diagnostics and environmental monitoring, with pilot deployments expected in the near term.
Looking forward, the outlook for graphane-based membrane engineering is closely tied to advances in scalable manufacturing and cost reduction. As fabrication methods mature and industry partnerships deepen, commercialization across filtration, energy storage, and sensing is likely to accelerate, positioning graphane membranes as a key material platform for the next wave of high-performance, sustainable technologies.
Global Market Forecasts and Revenue Projections (2025–2030)
As of 2025, graphane-based membrane engineering stands at a pivotal moment, with the global market poised for significant expansion over the coming five years. The unique properties of graphane—such as its exceptional mechanical strength, chemical stability, and tunable band gap—have positioned it as a promising candidate for next-generation membrane technologies in sectors ranging from water desalination and gas separation to advanced energy storage and biomedical devices.
Recent collaborations between materials manufacturers and academic institutions have accelerated the scaling of graphane membrane production. For instance, major companies in the advanced materials sector—including 2D Materials Pte Ltd and Graphenea—have reported ongoing investment in pilot-scale synthesis and integration techniques for graphane and related two-dimensional materials. These developments are expected to translate into commercial deployments by 2026–2027, especially in applications demanding ultra-high selectivity and molecular permeability.
Revenue projections for graphane-based membrane technologies are robust. Industry analysts anticipate that the global market for advanced nanomaterial membranes could reach the lower single-digit billions of USD by 2030, with graphane-based products capturing a growing share as manufacturing processes mature. Key growth drivers include the rising demand for energy-efficient separation technologies, regulatory emphasis on clean water access, and the rapid electrification of industrial processes. Notably, Henkel AG & Co. KGaA and BASF SE have both cited ongoing R&D into carbon-based membranes, potentially incorporating graphane, as part of their next-generation filtration and separation product lines.
Regionally, Asia-Pacific is expected to lead adoption, especially in countries investing heavily in water infrastructure and green hydrogen production. European and North American players are also scaling up, focusing on specialty niches such as pharmaceutical purification and advanced battery technologies. Strategic alliances and public-private partnerships will play a vital role in mitigating the high initial costs of graphane membrane manufacturing, further accelerating market penetration.
Looking ahead to 2030, industry outlooks forecast a compound annual growth rate (CAGR) in the double digits for graphane-based membrane solutions, outpacing traditional polymer and single-layer graphene membranes. As process scalability improves and costs decrease, graphane membranes are likely to become a mainstay in global separation and filtration markets, underpinning advances in clean energy, healthcare, and environmental remediation.
Competitive Analysis: Leading Companies and Innovators
The market for graphane-based membrane engineering is poised for significant activity in 2025, as a select group of companies and innovators intensify their efforts to commercialize this advanced material for filtration, separation, and energy applications. As of 2025, the landscape is characterized by early-stage product launches, pilot-scale manufacturing, and strategic collaborations between research institutes and industry leaders. This section analyzes the competitive positioning of key players actively developing graphane membrane technologies.
Among the foremost innovators is Graphenea, a Spain-based graphene materials manufacturer. While primarily known for its high-quality graphene, the company has expanded its research and development portfolio to encompass hydrogenated derivatives, including graphane. Their collaborative projects with European research consortia have demonstrated promising results in fabricating atomically-thin graphane membranes with enhanced selectivity for gas separation and water purification. The company’s vertically integrated approach—from synthesis to device integration—offers it a competitive edge as demand for next-generation filtration materials grows.
Another key contender is Directa Plus, an Italian specialist in advanced graphene-based solutions. Directa Plus has invested in membrane engineering through partnerships with engineering firms and water utilities, aiming to translate laboratory-scale graphane membrane prototypes into scalable products. In 2025, their pilot projects focus on leveraging graphane’s tunable permeability for desalination and industrial wastewater treatment, targeting both European and Middle Eastern markets.
On the academic–industry front, Massachusetts Institute of Technology (MIT) remains a notable driver of innovation. MIT’s research groups have published breakthrough results on the fabrication and functionalization of graphane membranes for selective ion transport and hydrogen storage. Their ongoing partnerships with multinationals in the chemical and energy sectors signal potential technology transfer opportunities and commercialization pathways within the next few years.
Additionally, Samsung Electronics has filed patents and participated in consortia focused on two-dimensional materials, including graphane, for use in advanced filtration and electronics cooling systems. The company’s global reach and manufacturing infrastructure position it as a potential large-scale adopter should pilot projects prove economically viable.
Looking ahead, the competitive environment is expected to intensify as performance data from pilot installations become available and regulatory frameworks for nanomaterial-based membranes evolve. Early movers with scalable production capabilities, robust intellectual property portfolios, and strong industry partnerships are likely to shape the trajectory of graphane-based membrane engineering through 2025 and beyond.
Technological Challenges and R&D Frontiers
Graphane-based membrane engineering has emerged as a dynamic research frontier, driven by the unique properties of graphane—namely its tunable bandgap, chemical stability, and potential for selective permeability. However, as of 2025, several technological challenges continue to shape the landscape of research and development (R&D) in this field.
A primary challenge is the scalable synthesis of defect-free graphane films with uniform hydrogenation. Controlled hydrogenation at the atomic level remains difficult, leading to variability in membrane performance. Researchers are currently investigating plasma-enhanced chemical vapor deposition (PECVD) and advanced atomic layer deposition (ALD) as promising routes to achieve consistent and large-area graphane films. Early-stage collaborations between academic institutions and industry players, such as those involving Samsung Electronics and BASF, are exploring these manufacturing pathways, though industrial-scale adoption is still in the pilot phase.
Another R&D barrier is the integration of graphane membranes into existing separation and filtration systems. Unlike graphene oxide or polymeric membranes, graphane’s inertness and hydrophobicity require innovative surface functionalization techniques to tailor selectivity for water purification or gas separation. Companies like Dow and Evonik Industries are investing in chemical modification approaches, such as introducing heteroatom dopants or patterned hydrogenation, to enhance compatibility and performance.
Mechanical robustness and long-term stability under operational conditions are also under active investigation. Graphane membranes tend to suffer from hydrogen desorption when exposed to high temperatures or aggressive chemicals, which can compromise structural integrity and separation efficacy. R&D efforts in 2025 are focusing on composite architectures—embedding graphane within polymer matrices or sandwiching it between protective layers—to address these durability concerns. Pilot testing by manufacturers, including SUEZ, is assessing these hybrid designs in industrial wastewater treatment and gas processing scenarios.
Looking ahead, the next several years are expected to witness progress in three key areas: (1) improved synthesis techniques for large-area, defect-minimized graphane membranes; (2) scalable integration with established membrane modules; and (3) comprehensive lifecycle analyses to validate commercial viability and environmental impact. The momentum in R&D, coupled with growing interest from chemical and water technology sectors, suggests that graphane-based membranes may transition from laboratory-scale prototypes to early commercial demonstration by the late 2020s, provided current challenges are systematically addressed.
Regulatory and Standards Outlook
The regulatory landscape for graphane-based membrane engineering is expected to evolve rapidly through 2025 and the following years, reflecting both the novelty of the material and the rising interest in advanced membrane technologies across industries such as water treatment, electronics, and energy. Unlike graphene, which has enjoyed growing regulatory clarity since its commercial adoption, graphane—a hydrogenated derivative of graphene—remains in the early stages of regulatory scrutiny. However, as research transitions to pilot-scale demonstrations and early commercialization, especially in filtration and separation applications, regulatory bodies are beginning to address safety, environmental, and performance standards tailored to these novel materials.
In the European Union, regulatory attention is likely to align with the framework developed for nanomaterials, particularly through the European Chemicals Agency (European Chemicals Agency). As graphane-based membranes often qualify as nanomaterials under REACH regulations, manufacturers are expected to provide detailed dossiers on physical-chemical properties, toxicological profiles, and environmental impact assessments prior to market introduction. The European Commission is also supporting standardization work in this area, aiming to harmonize testing and certification protocols for novel carbon-based membranes.
In the United States, oversight is likely to be shared between the U.S. Environmental Protection Agency—especially for water treatment applications—and agencies such as the U.S. Food and Drug Administration if graphane-based membranes are used in medical devices or food contact materials. Recent statements from the National Electrical Manufacturers Association have highlighted the need for clear safety and performance standards as electrically integrated membranes, including those using graphane, enter the market.
Internationally, the International Organization for Standardization is expected to play a key role in developing technical standards for characterization, mechanical integrity, and lifecycle assessment of graphane-based membranes. Ongoing work under ISO/TC 229 (Nanotechnologies) and ISO/TC 282 (Water Reuse) will likely expand to explicitly include graphane derivatives as their market presence grows.
Looking ahead, the next few years should see pilot regulatory frameworks and voluntary standards that address not only the unique properties of graphane but also the need for traceability, recyclability, and occupational safety in manufacturing environments. Collaboration between industry consortia and standards bodies, such as those coordinated by ASTM International, is anticipated to accelerate the safe and responsible adoption of graphane-based membrane technologies globally.
Sustainability and Environmental Impact
Graphane-based membrane engineering is emerging as a promising field for sustainable separations, water purification, and environmental remediation. As of 2025, the unique properties of graphane—hydrogenated graphene with a tunable bandgap, high chemical stability, and atomically-thin structure—are being harnessed to address pressing challenges in resource efficiency and ecological impact.
Recent advances have focused on synthesizing large-area, defect-controlled graphane membranes with high selectivity and permeability. Researchers are reporting that, compared to traditional polymeric and ceramic membranes, graphane’s precisely tunable nanopores can facilitate ultrafast water transport while blocking contaminants such as heavy metals and organic pollutants. Early prototypes demonstrate high rejection rates (>99%) and fluxes several times higher than commercial alternatives, holding potential for energy savings and reduced material consumption.
Industrial interest is intensifying. Companies involved in membrane technology and advanced materials, such as SUEZ and DuPont, are exploring graphene-derivative membranes, and are expected to trial graphane-based variants as the synthesis becomes more scalable. These organizations are motivated by stricter regulatory environments and the demand for greener solutions in both municipal and industrial water treatment. Simultaneously, suppliers like Graphenea are ramping up production capabilities for custom graphene derivatives, including graphane, to meet anticipated market needs.
From a life cycle perspective, the environmental footprint of graphane-based membranes is projected to be lower than that of conventional materials—mainly due to their longer operational lifetimes, reduced fouling, and lower energy requirements for operation. Initial data from pilot installations indicate up to 30% reductions in operational energy use and significant decreases in chemical cleaning frequency compared to legacy membranes, contributing to lower greenhouse gas emissions and less chemical waste.
Looking ahead, the next few years will likely see further integration of graphane membranes in pilot-scale water treatment, desalination, and gas separation facilities. Collaboration between academic research centers, material suppliers, and major water technology firms is expected to accelerate commercialization. However, full-scale adoption will depend on continued improvements in cost-effective synthesis, robust membrane module design, and detailed environmental impact assessments—areas that are top priorities for industry stakeholders through 2025 and beyond.
Strategic Partnerships, Investments, and M&A Trends
The landscape for strategic partnerships, investments, and mergers & acquisitions (M&A) in the field of graphane-based membrane engineering is poised for significant evolution in 2025 and the ensuing years. Driven by the promise of superior mechanical, electrical, and selective permeability properties, graphane-based membranes are attracting increased interest from established industry players and innovative startups alike.
A notable trend in 2025 is the emergence of cross-sector alliances between advanced materials companies and major players in the water treatment, energy, and electronics industries. For example, established membrane manufacturers such as Toray Industries, Inc. and Evonik Industries AG have expanded their innovation programs to include two-dimensional (2D) materials, including graphane derivatives, seeking to capitalize on their potential for next-generation filtration and separation technologies. Such strategic collaborations are often structured as joint R&D agreements, with shared access to pilot-scale facilities and IP portfolios.
In parallel, leading chemical and advanced materials suppliers, including BASF SE, are actively investing in startups and university spin-offs focused on scalable graphane synthesis and membrane fabrication. These investments typically take the form of equity stakes, licensing agreements, or direct funding of proof-of-concept projects. In 2025, an uptick is anticipated in seed and Series A investment rounds for early-stage companies demonstrating processability breakthroughs, such as continuous roll-to-roll graphane membrane production, which is critical for commercial adoption.
M&A activity is also forecast to intensify as larger corporations seek to secure a technological edge and accelerate their entry into the graphane membrane market. Companies with existing graphene and other 2D-material portfolios, such as Versarien plc and Directa Plus plc, are considered likely candidates for acquiring or merging with firms specializing in graphane functionalization and application-specific engineering. These moves are expected to consolidate IP, streamline scale-up efforts, and facilitate rapid integration into end-use sectors such as desalination, hydrogen production, and specialty electronics.
Looking ahead, industry observers anticipate that strategic partnerships will increasingly focus on co-developing application-tailored graphane membranes, particularly for water purification and energy storage. Furthermore, as regulatory frameworks mature and standardization efforts led by organizations like International Organization for Standardization advance, additional capital inflows and cross-border collaborations are projected. Overall, 2025 is set to be a pivotal year for commercial and strategic activity in graphane-based membrane engineering, setting the stage for broader adoption in the latter half of the decade.
Future Outlook: Emerging Opportunities and Disruptive Potential
The field of graphane-based membrane engineering is poised for substantial advancements in 2025 and the coming years, driven by the material’s unique structural and electronic properties. Graphane, a fully hydrogenated derivative of graphene, offers tunable band gaps, high thermal stability, and chemical inertness—characteristics that are opening new frontiers in membrane technology for separation, filtration, and energy applications.
One significant emerging opportunity lies in the development of next-generation water purification and desalination membranes. Research efforts in 2024 demonstrated that graphane’s atomic-scale pores can be precisely engineered to allow rapid water transport while effectively excluding salts and contaminants. This positions graphane membranes as potential game-changers for municipal and industrial water treatment, offering both high selectivity and robust mechanical performance. Companies specializing in advanced filtration solutions and materials integration, such as Dow and Toray Industries, are likely to accelerate prototype development and scale-up in collaboration with academic and government laboratories.
In gas separation, graphane’s variable hydrogenation patterns enable the tailoring of pore sizes and surface chemistry, which is crucial for selective CO2, H2, and N2 separations. The rising focus on carbon capture and hydrogen purification, especially within the energy transition strategies of major chemical and energy sector players, is expected to drive collaborative R&D. Organizations like Shell and Air Liquide have expressed interest in advanced membrane technologies for process intensification and emissions reduction, making them potential early adopters of graphane-based solutions.
The disruptive potential of graphane membranes extends to solid-state energy storage and conversion devices. The integration of graphane layers as protective or selective interfaces in fuel cells and lithium-ion batteries is under early investigation, with the aim to enhance ionic conductivity while preventing dendrite formation and side reactions. Companies such as Samsung, known for their investment in advanced materials for batteries, are monitoring these developments for incorporation into next-generation energy storage platforms.
Looking ahead, challenges remain in the cost-effective, scalable synthesis of defect-free graphane sheets and the reliable fabrication of large-area membranes. However, the rapid progress in chemical vapor deposition (CVD) and atomic layer deposition (ALD) techniques, alongside increasing industrial interest and public funding initiatives, suggest that commercial deployment of graphane-based membranes could begin within the latter half of the decade. As industry standards evolve and pilot projects mature, graphane membranes are positioned to disrupt traditional markets in filtration, gas separation, and energy, marking a transformative period for membrane engineering.
Sources & References
- Directa Plus
- First Graphene
- SUEZ
- Toray Industries
- DuPont
- Toshiba Corporation
- Oxford Instruments
- Henkel AG & Co. KGaA
- BASF SE
- Massachusetts Institute of Technology (MIT)
- Evonik Industries
- European Chemicals Agency
- European Commission
- National Electrical Manufacturers Association
- International Organization for Standardization
- ASTM International
- Versarien plc
- Shell
- Air Liquide