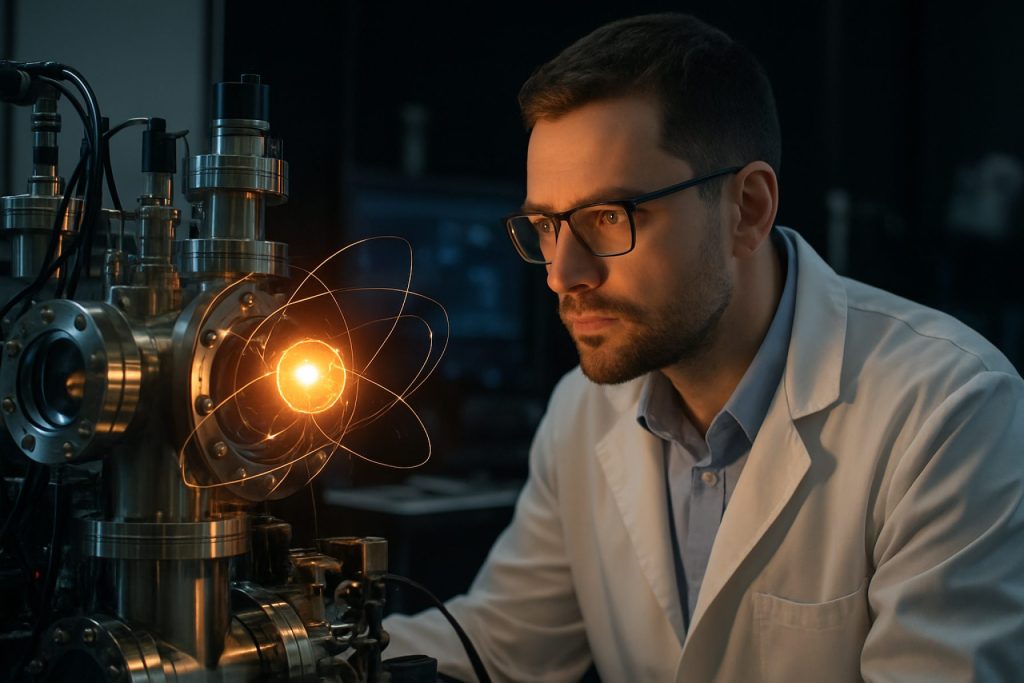
How Quasixenon Particle Spectroscopy is Rewriting the Rules of Advanced Materials and Physics in 2025: Breakthroughs, Market Leaders, and the Surprising Future Unveiled
- Executive Summary: 2025 Market at a Glance
- Technology Overview: Fundamentals & Innovations in Quasixenon Particle Spectroscopy
- Competitive Landscape: Key Players and Strategic Alliances (e.g., ieee.org, oxinst.com)
- Current Market Size & Segmentation by Application
- Major 2025-2029 Growth Drivers and Restraints
- Emerging Use Cases in Quantum Computing and Nanomaterials
- Investment Trends and Funding Outlook
- Breakthroughs in Instrumentation and Data Analytics
- Regulatory Landscape and Industry Standards (e.g., ieee.org)
- Future Outlook: Market Forecasts and Disruptive Opportunities Through 2030
- Sources & References
Executive Summary: 2025 Market at a Glance
Quasixenon Particle Spectroscopy (QPS) has rapidly transitioned from a specialized analytical technique to a pivotal tool in advanced materials research, environmental monitoring, and next-generation semiconductor manufacturing. In 2025, the global market for QPS solutions is witnessing robust growth, underpinned by technological innovation, expanding application domains, and rising demand for ultra-sensitive particle detection. Major equipment manufacturers, including Thermo Fisher Scientific and Carl Zeiss AG, have launched new QPS platforms featuring enhanced sensitivity, real-time analytics, and integration with AI-driven data interpretation—catering to the needs of both academic and industrial laboratories.
Key events shaping the 2025 landscape include increased standardization of QPS methodologies, with collaborations spearheaded by leading industry bodies such as the SEMI association to develop reference protocols for semiconductor defect analysis. The past year has seen significant adoption of QPS instruments by major chip manufacturers, notably in Asia-Pacific and North America, to address sub-nanometer contamination challenges in EUV lithography and advanced packaging. Hitachi High-Tech Corporation and JEOL Ltd. have expanded their product lines with QPS-enabled electron microscopy systems, targeting both research and high-throughput inspection markets.
Data from industry sources indicate that QPS instrumentation sales have surpassed expectations in 2024, with double-digit percentage growth forecast through 2026. Environmental monitoring agencies are also deploying QPS for trace-level detection of xenon isotopes, a critical need in nuclear non-proliferation and climate science. Companies such as Bruker Corporation are collaborating with governmental organizations to tailor QPS solutions for real-time atmospheric sampling and analysis.
Looking ahead, the outlook for QPS in 2025 and beyond is characterized by continued miniaturization of detection hardware, wider adoption in pharmaceutical quality control, and ongoing integration with cloud-based data platforms. The entry of new regional manufacturers, particularly in South Korea and Germany, is expected to intensify competition and drive further innovation. Strategic investments by established players—including expanded service and training networks by Thermo Fisher Scientific—will likely cement QPS as an essential technology across multiple high-impact sectors.
Technology Overview: Fundamentals & Innovations in Quasixenon Particle Spectroscopy
Quasixenon Particle Spectroscopy (QXPS) represents a frontier analytical technique rooted in the detection and analysis of exotic quasiparticles in noble gas matrices, particularly xenon. Building on the established legacy of xenon-based detectors in rare event searches and particle physics, QXPS leverages the unique interaction cross-sections and high purity achievable with xenon to probe subatomic processes with unprecedented sensitivity. The key principle involves the excitation and subsequent relaxation of quasixenon states, which can arise through interactions with incident particles, enabling precise energy resolution and particle identification capabilities.
Recent years have witnessed significant advances in both the theoretical framework and practical instrumentation underpinning QXPS. In 2025, detector manufacturers are focusing on integrating ultra-low-background materials and improved photodetection arrays to reduce noise and increase quantum efficiency. Innovations in cryogenic cooling and gas purification, critical for maintaining xenon purity and stability, are being spearheaded by technology leaders such as Linde, renowned for their expertise in rare gas supply and handling, and Air Liquide, which provides specialized xenon isotopic enrichment and purification solutions. These companies are collaborating with research institutions to develop next-generation xenon handling systems suitable for large-scale, high-sensitivity QXPS setups.
At the detector level, companies like Hamamatsu Photonics have advanced the development of silicon photomultipliers and vacuum photomultiplier tubes capable of resolving single-photon events within the deep ultraviolet spectrum characteristic of xenon scintillation. Their ongoing R&D is focused on enhancing photon detection efficiency and reducing intrinsic radioactivity, directly impacting the sensitivity and accuracy of QXPS detectors.
A notable trend in 2025 is the miniaturization and ruggedization of QXPS instrumentation, enabling its deployment in field settings beyond traditional laboratory environments. This is facilitated by modular detector architectures and robust electronics developed by instrumentation firms such as Teledyne Technologies, which support rapid data acquisition and real-time spectral analysis. The integration of machine learning algorithms for pattern recognition and background discrimination is also becoming a standard feature, further improving analytical throughput and reliability.
Looking ahead, the outlook for QXPS is marked by extensive cross-disciplinary collaboration, driving both fundamental discovery and applied innovation. As the demand for trace-level detection in environmental monitoring, nuclear nonproliferation, and medical diagnostics grows, QXPS is poised to become a cornerstone technology, benefiting from ongoing advances in materials science, photodetection, and data analytics. The presence of established suppliers and technology enablers, actively investing in R&D and large-scale production capabilities, ensures a robust innovation pipeline for the coming years.
Competitive Landscape: Key Players and Strategic Alliances (e.g., ieee.org, oxinst.com)
The competitive landscape for Quasixenon Particle Spectroscopy (QPS) in 2025 is defined by a small cadre of advanced instrumentation manufacturers, specialized component suppliers, and strategic research alliances. As QPS technology matures and finds broader applications in fields such as nuclear physics, environmental monitoring, and materials science, the sector is witnessing both consolidation and innovation among key players.
Among the most prominent commercial entities, Oxford Instruments stands out for its portfolio of high-performance spectrometers and particle detection systems. The company has invested significantly in developing modular platforms and software suites that enable real-time analysis of rare-gas isotope spectra, including specialized systems tailored for quasixenon detection. These advances are underpinned by collaborations with European nuclear research agencies and universities, accelerating the transition of QPS tools from prototype to commercial viability.
In the US, Thermo Fisher Scientific is leveraging its established expertise in analytical instrumentation to expand into QPS. Recent product launches in 2024 and early 2025 include upgrades to their mass spectrometry lines, incorporating xenon-compatible ion sources and enhanced detection modules. Strategic alliances with particle accelerator facilities are facilitating the validation of these technologies in real-world research environments.
On the component side, Hamamatsu Photonics plays a crucial role as a supplier of photomultiplier tubes (PMTs) and silicon photomultipliers (SiPMs), both integral to next-generation QPS detectors. Hamamatsu’s focus on low-background, high-sensitivity photon detection aligns with the needs of quasixenon measurement, particularly for rare-event searches and ultra-trace analysis.
Industry consortia and standards bodies are also shaping the landscape. The Institute of Electrical and Electronics Engineers (IEEE) continues to facilitate interoperability and data-exchange standards for particle spectroscopy instrumentation, which is critical as multi-vendor systems become more common in advanced laboratories. The IEEE’s Nuclear and Plasma Sciences Society regularly convenes working groups and technical panels to develop best practices for QPS deployment and data management.
Looking ahead to the next few years, strategic alliances are expected to intensify, particularly between academic research hubs and industrial manufacturers. Joint ventures focused on miniaturization, automation, and AI-driven spectral analysis are already in development, with pilot projects scheduled through 2026. As detection thresholds improve and regulatory frameworks mature, the competitive landscape will likely see new entrants from adjacent markets, such as semiconductor metrology and environmental monitoring, further spurring innovation and adoption of QPS technologies.
Current Market Size & Segmentation by Application
Quasixenon Particle Spectroscopy (QPS) is an emerging analytical technology occupying a niche in advanced materials characterization, semiconductor fabrication, and environmental monitoring. As of 2025, the QPS market is still in a nascent phase, but it is gaining traction due to its ability to provide high-sensitivity detection and precise measurement of xenon-tagged particles across varied matrices.
The global market size for QPS instrumentation and related services is estimated to be in the low hundreds of millions USD as of early 2025. This relatively modest figure reflects both the recent commercialization of core QPS systems and the ongoing process of technical validation across multiple industries. The market is primarily driven by increasing demand for ultraprecise particle identification—particularly in sectors where xenon isotopic tracing offers clear advantages over conventional mass spectrometry or optical methods.
- Semiconductor Manufacturing: The largest and fastest-growing application segment for QPS in 2025 is semiconductor process monitoring. Leading chipmakers and foundries are leveraging QPS to detect trace levels of xenon-tagged contamination, inform process optimization, and validate advanced lithography steps. Companies such as Applied Materials and Lam Research are pioneering the integration of QPS modules into existing metrology platforms, enhancing yield management in sub-3nm device fabrication.
- Materials Science: Academic and industrial laboratories are adopting QPS for the analysis of advanced nanomaterials and heterostructures, where xenon isotopic labeling facilitates the study of diffusion, aggregation, or degradation pathways at unprecedented resolution. Instrument makers like Oxford Instruments and JEOL Ltd. have announced pilot programs and beta releases of QPS-enabled particle analyzers tailored for research environments.
- Environmental Monitoring: QPS is being evaluated by environmental agencies and select industrial consortia for monitoring xenon-tagged tracer particles in air and water. This application is expected to see moderate growth through 2028, especially as regulatory frameworks evolve to require finer granularity in pollutant source tracing.
- Healthcare and Life Sciences: Early-stage research collaborations are exploring the use of QPS for tracking xenon-labeled drug delivery vehicles and diagnostic agents in preclinical trials. While this segment is not yet commercialized, partnerships between instrument suppliers and pharmaceutical companies are ongoing.
Looking ahead, the QPS market is projected to diversify as more industries validate the technology’s benefits. The sector’s expansion will depend on continued innovation by manufacturers, including Bruker Corporation, and broader adoption of xenon labeling protocols. As cost barriers decrease and regulatory acceptance grows, QPS is poised for double-digit annual growth in both instrumentation and service segments through the late 2020s.
Major 2025-2029 Growth Drivers and Restraints
Quasixenon Particle Spectroscopy (QPS) stands at the forefront of advanced analytical technologies for high-sensitivity particle detection and characterization, with its applications spanning materials science, semiconductor fabrication, environmental monitoring, and nuclear research. As of 2025, several factors are expected to drive significant growth in the QPS sector through to 2029, alongside notable restraints shaping industry development.
Major Growth Drivers (2025–2029):
- Rising Demand for Ultra-Trace Particle Analysis: The miniaturization of semiconductor devices and the evolution of advanced materials require increasingly precise detection of contaminants at the sub-nanometer scale. QPS technologies, leveraging the unique properties of quasixenon, offer enhanced sensitivity and selectivity, enabling leading manufacturers to ensure ultra-pure production environments. Companies such as HORIBA and Thermo Fisher Scientific are key innovators, integrating QPS modules into their analytical platforms to meet these stringent requirements.
- Growth in Nuclear and Environmental Monitoring: Regulatory tightening on radioactive particle release and the need for accurate environmental monitoring have accelerated the adoption of QPS, especially for xenon isotope detection—a critical indicator in nuclear treaty verification and atmospheric studies. Organizations such as International Atomic Energy Agency are expected to further invest in QPS-based surveillance infrastructure over the coming years.
- Expansion of R&D Initiatives: Governments and industry leaders are increasing investments in QPS research to explore next-generation detectors and portable field-deployable systems. Collaborative projects between national laboratories and instrumentation suppliers, including Bruker and Agilent Technologies, are accelerating the pace of innovation, fostering a robust pipeline of new applications.
Key Restraints:
- High Initial Investment and Technical Complexity: The capital expenditure associated with QPS systems remains substantial, particularly due to the specialized xenon gas handling, calibration, and maintenance requirements. This can pose adoption challenges for smaller laboratories and resource-limited regions.
- Skilled Workforce Shortage: The operation and interpretation of QPS data demand advanced training. The current shortage of skilled personnel, especially outside major research hubs, may limit the broader deployment of these systems.
- Regulatory and Supply Chain Constraints: The procurement of high-purity xenon and compliance with international transport regulations could impact the operational scalability of QPS platforms, particularly as global supply chains remain sensitive to geopolitical developments.
Outlook: Between 2025 and 2029, the QPS sector is poised for robust expansion, driven by semiconductor, nuclear, and environmental market demands. Overcoming cost barriers, workforce gaps, and supply chain vulnerabilities will be critical for manufacturers such as HORIBA, Thermo Fisher Scientific, and their counterparts as they shape the next phase of analytical instrumentation evolution.
Emerging Use Cases in Quantum Computing and Nanomaterials
Quasixenon particle spectroscopy, an advanced analytical technique utilizing quasi-stable xenon isotopes, is rapidly gaining traction for its unique sensitivity and selectivity in probing nanomaterials and quantum systems. As of 2025, a wave of new use cases is emerging, particularly driven by the evolving demands of quantum computing and the characterization of next-generation nanomaterials.
Quantum computing hardware developers are increasingly leveraging quasixenon particle spectroscopy to investigate material defects, coherence properties, and impurity distributions at the atomic scale. This is especially critical for superconducting qubits and topological quantum devices, where minute imperfections can severely impact device performance. Industry leaders such as IBM and Google are known for their in-house research on quantum hardware, focusing on ultra-pure materials and precise atomic engineering. While these companies do not publicly detail their specific spectroscopy methodologies, supplier partnerships and academic collaborations suggest that advanced rare-gas spectroscopies, including quasixenon-based approaches, are in experimental use to optimize device yields and coherence times.
In the nanomaterials sector, quasixenon particle spectroscopy is being adopted for the non-destructive analysis of two-dimensional materials, quantum dots, and heterostructures. The method’s ability to resolve trace contaminants and subtle lattice distortions is valuable for manufacturers of semiconductor and optoelectronic components. Companies such as ASM International, a major supplier of atom-scale metrology equipment, are actively developing instrumentation platforms that could integrate quasixenon-based techniques, aiming to meet the increasingly stringent requirements of atomic-layer manufacturing.
Collaboration between metrology equipment manufacturers and specialty gas suppliers is also accelerating. Firms like Air Liquide and Linde—global leaders in the production and purification of noble gases—are investing in the supply chains required for high-purity xenon isotopes, anticipating a surge in demand from quantum and nanotech sectors. These efforts are further supported by national laboratories and standards institutes, which are working to establish reference materials and measurement protocols suitable for the high precision required in quantum applications.
Looking ahead, the next few years are expected to see broader commercial deployment of quasixenon particle spectroscopy in both industrial and academic R&D settings. Key challenges include reducing the cost of enriched xenon isotopes and improving the integration of spectroscopy platforms into existing fabrication lines. However, with major stakeholders across the value chain—from quantum computing pioneers to process equipment giants and gas suppliers—committed to advancing the technology, quasixenon particle spectroscopy is poised to become a foundational tool for the next generation of quantum and nanomaterial innovations.
Investment Trends and Funding Outlook
Quasixenon particle spectroscopy, a leading-edge analytical technique for detecting and characterizing subatomic phenomena, has seen a notable uptick in investment activity as global interest in advanced materials, quantum research, and high-energy physics intensifies. The funding landscape in 2025 is shaped primarily by the interplay between government research initiatives, private sector innovation, and strategic alliances with equipment manufacturers.
Significant public investment continues to flow from large-scale national and supranational research programs. Agencies such as the European Organization for Nuclear Research (CERN) remain central, with multi-year commitments to upgrading detection infrastructure and particle accelerators. CERN’s inclusion of quasixenon-based technologies in its particle identification roadmaps has triggered parallel funding from the European Union’s Horizon Europe framework and national science bodies. In the United States, the U.S. Department of Energy is maintaining grant support for university laboratories at the forefront of next-generation spectroscopy, with allocations specifically earmarked for xenon and quasi-xenon detector development.
On the industry side, manufacturers specializing in high-purity gases and detection equipment are responding to research demand with targeted investment in R&D. Linde and Air Liquide, both global leaders in rare gas supply, have announced expanded production capabilities for isotopes and ultra-pure xenon mixtures, supporting not only scientific instrumentation but also related medical and semiconductor markets. Meanwhile, detector specialists like Hamamatsu Photonics and Carl Zeiss AG are scaling up the development of novel photodetectors and readout electronics tailored for quasixenon applications.
Venture capital involvement, though modest compared to government funding, is growing, particularly in North America, where early-stage companies are commercializing portable quasixenon spectrometers for industrial and security markets. Notably, partnerships between equipment suppliers and university spinouts have attracted seed investment, with several consortia forming to accelerate technology transfer.
Looking ahead to the next few years, the funding outlook for quasixenon particle spectroscopy remains robust. The convergence of increased government research budgets, industry’s drive to support precision measurement, and the gradual emergence of commercial applications is expected to sustain a positive investment climate. Key developments to watch include expanded participation from Asia-Pacific research institutes and suppliers—particularly as entities like Mitsubishi Electric Corporation and Hitachi, Ltd. deepen their involvement in quantum and particle detection systems.
Breakthroughs in Instrumentation and Data Analytics
Quasixenon Particle Spectroscopy (QPS) has rapidly advanced in recent years, propelled by breakthroughs in both instrumentation and data analytics. As of 2025, industry leaders are leveraging enhanced xenon-based detection systems and state-of-the-art digital signal processing to push the boundaries of single-particle sensitivity and selectivity. These advancements are particularly relevant for applications in nuclear security, rare event searches, and advanced materials characterization.
Instrumentation breakthroughs have centered around the integration of ultrahigh-purity xenon targets with cryogenic and time-projection chamber (TPC) technologies. Companies such as Honeywell and Thermo Fisher Scientific are actively developing systems that combine precise xenon gas handling with real-time, high-throughput particle detection. These modular units facilitate rapid deployment in both laboratory and field settings, with advances in photodetector arrays and low-noise electronics yielding improved discrimination between quasixenon events and background signals.
On the analytical side, the adoption of machine learning and advanced statistical modeling has yielded substantial gains in data interpretation. Multi-modal data fusion techniques, integrating calorimetric, timing, and spatial event data, are now routinely implemented to enhance sensitivity to rare particle interactions. Carl Zeiss AG, while traditionally focused on optics and microscopy, has entered the particle spectroscopy arena with proprietary AI-driven data analytics platforms designed to facilitate automated event classification and real-time anomaly detection.
Collaboration between industry and public research institutions remains a key driver. Initiatives funded through partnerships with organizations like Oak Ridge National Laboratory and Brookhaven National Laboratory are accelerating the development of next-generation QPS systems. These efforts are expected to yield detectors with unprecedented resolution and scalability, aimed at both fundamental research and emerging commercial markets.
Looking ahead to the next few years, the outlook for QPS is robust. Industry stakeholders are prioritizing the miniaturization of detector systems and the integration of cloud-based analytics for remote monitoring and collaborative research. Roadmaps published by sector leaders anticipate the deployment of portable QPS devices in environmental monitoring, medical diagnostics, and even quantum technology validation by 2027. As QPS becomes increasingly accessible and automated, its role in both scientific discovery and applied sectors is set to expand considerably.
Regulatory Landscape and Industry Standards (e.g., ieee.org)
The regulatory landscape for Quasixenon Particle Spectroscopy (QPS) is evolving rapidly as its applications expand in fields such as semiconductor manufacturing, nuclear detection, and advanced materials research. As of 2025, industry and governmental bodies are actively working to update and harmonize standards to ensure accuracy, interoperability, and safety in the deployment of QPS technologies.
On the international stage, the Institute of Electrical and Electronics Engineers (IEEE) has initiated working groups focused on standardizing QPS instrument calibration, data reporting formats, and electromagnetic compatibility. The IEEE’s standardization process is particularly influential in ensuring that QPS devices can be integrated into larger analytical systems and that their results are comparable across laboratories worldwide.
In the United States, the National Institute of Standards and Technology (NIST) plays a central role in metrological traceability for QPS. In 2025, NIST is collaborating with major instrument manufacturers and research consortia to develop reference materials and protocols for quasixenon-based particle detection, aimed at supporting accreditation and regulatory compliance in both academic and industrial settings. This includes efforts to define performance benchmarks such as detection limits, energy resolution, and particle discrimination capabilities.
The European Union is also prioritizing updates to its analytical instrumentation directives. Organizations like CEN-CENELEC are working with manufacturers to address regulatory requirements for QPS use in sensitive environments, particularly in the context of environmental monitoring and radiation safety. New guidance documents and conformity assessment frameworks specific to QPS are expected to be published in late 2025, reflecting the technology’s growing role in critical infrastructure monitoring.
On the industry side, leading QPS instrument manufacturers are proactively engaging with standards bodies to ensure compatibility with forthcoming regulations. For example, Thermo Fisher Scientific and Hitachi have announced partnerships with regulatory agencies to develop and validate test procedures for their next-generation QPS platforms. These efforts are intended to streamline certification processes and facilitate market access for their products in regions with evolving regulatory demands.
Looking ahead, increased regulatory clarity and harmonization are anticipated to accelerate adoption of QPS in high-reliability sectors, such as aerospace, nuclear energy, and pharmaceuticals. Industry stakeholders expect that by 2027, unified global standards will reduce compliance costs and enable broader interoperability, further fueling innovation and deployment of QPS technologies.
Future Outlook: Market Forecasts and Disruptive Opportunities Through 2030
Quasixenon Particle Spectroscopy (QPS) is set to undergo significant advancements and market expansion through 2030, driven by increasing demand for high-precision analytics in materials science, nuclear forensics, and advanced manufacturing. As of 2025, the sector is characterized by accelerating R&D investments and prototype deployments by key instrumentation manufacturers, with a growing emphasis on integrating QPS into semiconductor fabrication, environmental sensing, and particle physics research.
Instrument manufacturers specializing in spectroscopic and particle analysis technologies are leveraging quasixenon’s unique ionization and detection properties to develop next-generation detectors with enhanced sensitivity and reduced background noise. Companies such as Thermo Fisher Scientific and Bruker are actively expanding their advanced spectroscopy portfolios, with indications of ongoing research projects involving noble gas-based detection systems, including xenon and its analogs. Meanwhile, HORIBA continues to push innovation in particle characterization, exploring alternative carrier gases and detection chemistries appropriate for QPS applications.
Recent collaborations between research institutes and industry suggest that QPS platforms will be increasingly integrated into modular analytical workstations, enabling high-throughput, real-time analysis in both laboratory and industrial environments. The adaptability of QPS to both trace-level and bulk material characterization is attracting attention from sectors such as microelectronics—where sub-nanometer contamination control is critical—and nuclear safeguards, where the technique’s selectivity can aid in isotope fingerprinting.
From a market perspective, the next few years are expected to see increasing adoption of QPS-enabled systems in Asia-Pacific and North American markets, supported by national initiatives for advanced manufacturing and critical materials monitoring. Notably, several national laboratories and university consortia are partnering with leading vendors to validate QPS instrumentation in pilot-scale deployments, with the goal of establishing industry-wide detection standards.
By 2030, disruptive opportunities are anticipated in three main areas: (1) integration of QPS with artificial intelligence for automated spectral interpretation and anomaly detection; (2) miniaturization of QPS components for portable field use; and (3) the creation of hybrid multi-modal analysis systems combining QPS with complementary techniques such as Raman or X-ray fluorescence. Manufacturers with established expertise in high-purity noble gases, like Linde and Air Liquide, are expected to play a crucial role in ensuring the scalability and purity required for commercial QPS deployment.
In summary, Quasixenon Particle Spectroscopy is poised for substantial growth and technological disruption through 2030, supported by a robust ecosystem of instrument developers, gas suppliers, and end-user industries seeking unprecedented analytical performance.
Sources & References
- Thermo Fisher Scientific
- Carl Zeiss AG
- Hitachi High-Tech Corporation
- JEOL Ltd.
- Bruker Corporation
- Linde
- Air Liquide
- Hamamatsu Photonics
- Teledyne Technologies
- Oxford Instruments
- Institute of Electrical and Electronics Engineers (IEEE)
- HORIBA
- International Atomic Energy Agency
- IBM
- ASM International
- Air Liquide
- Linde
- European Organization for Nuclear Research (CERN)
- Mitsubishi Electric Corporation
- Hitachi, Ltd.
- Honeywell
- Oak Ridge National Laboratory
- Brookhaven National Laboratory
- National Institute of Standards and Technology (NIST)
- CEN-CENELEC