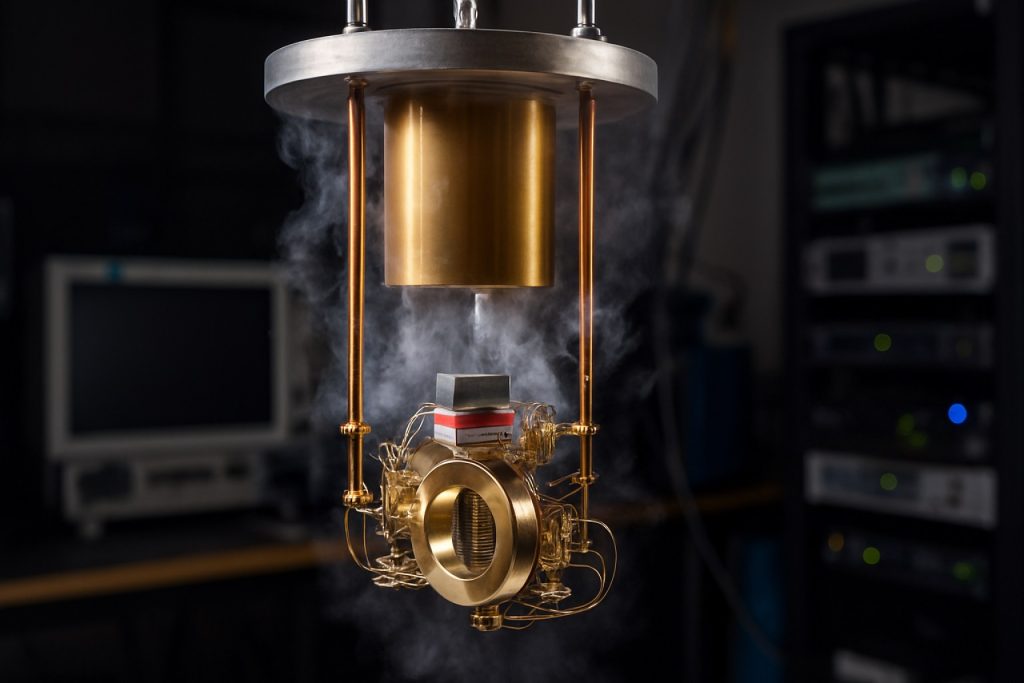
Table of Contents
- Executive Summary: 2025 Landscape and Key Takeaways
- Market Size & Forecast (2025–2030): Growth Trajectories and Demand Drivers
- Technology Evolution: From Lab to Real-World Cryogenic Spintronics
- Superconducting Materials: Innovations, Challenges, and Industry Leaders
- Quantum Computing Applications: Integrating Spintronics for Next-Gen Performance
- Data Storage and Memory: Cryogenic Spintronics as a Game Changer
- Competitive Landscape: Key Players and Strategic Moves (e.g., ibm.com, ieee.org)
- Regulatory, Standardization, and Ecosystem Developments (Refer to ieee.org)
- Investment, Partnerships, and M&A Activity Shaping the Sector
- Future Outlook: Emerging Opportunities and Disruptive Scenarios Through 2030
- Sources & References
Executive Summary: 2025 Landscape and Key Takeaways
Cryogenic superconducting spintronics is poised at a significant inflection point in 2025, characterized by notable advances in both materials science and device engineering. The field leverages the quantum properties of superconductors and the spin degree of freedom in electrons to enable ultra-efficient, high-speed, and low-power electronic components—key for next-generation computing, memory, and quantum information technologies.
Recent years have witnessed the transition of cryogenic superconducting spintronic devices from laboratory prototypes toward early-stage commercialization. Major manufacturers and government laboratories have demonstrated hybrid Josephson junctions and spin valves that operate at milli-Kelvin temperatures, showing promise for scalable cryogenic memory and logic circuits. This momentum is supported by the increased availability of high-quality thin films and multilayer heterostructures, especially those based on niobium and other low-temperature superconductors, from established suppliers such as Oxford Instruments and Kurt J. Lesker Company.
A notable driver in 2025 is the growing demand for quantum computing and high-performance cryogenic electronics. Industrial players such as IBM and Intel are actively exploring superconducting spintronic elements for integration into quantum processors and control systems, aiming to overcome the bottlenecks of conventional CMOS technologies at cryogenic temperatures. Similarly, collaborations with research organizations and national laboratories, including those coordinated by NIST, have accelerated the development of cryogenic magnetic random-access memory (Cryo-MRAM) and related logic devices.
In terms of practical deployment, the focus for the next few years will be on scaling up device integration, enhancing reproducibility, and tackling interface engineering challenges. Early demonstrators have achieved switching energies below 1 attojoule per bit and switching times in the sub-nanosecond regime, as evidenced by recent prototype reports from industry leaders and consortia. The path to commercialization will depend on further reduction in materials defects, reliable large-scale fabrication, and the development of compatible cryogenic packaging solutions—areas where suppliers like Bluefors and Cryomech are expected to play a crucial role.
In summary, the 2025 landscape for cryogenic superconducting spintronics is defined by growing industry investment, maturing supply chains, and a clear trajectory from proof-of-concept devices toward functional, scalable systems for quantum and high-performance computing. The next several years are likely to witness the first commercial deployments in specialized computing environments, paving the way for broader adoption as fabrication and integration hurdles are overcome.
Market Size & Forecast (2025–2030): Growth Trajectories and Demand Drivers
The cryogenic superconducting spintronics market is poised for significant growth between 2025 and 2030, driven by advances in quantum computing, high-performance computing (HPC), and ultra-sensitive sensing technologies. The demand for ultra-low power, high-speed logic and memory elements utilizing superconducting spintronic effects is accelerating as industry and government initiatives worldwide ramp up investments in next-generation computing infrastructure.
As of 2025, several leading organizations are scaling up research and pilot production of superconducting spintronic devices. For example, IBM continues to expand its quantum computing roadmap, with an eye toward integrating spintronics and superconducting circuits for improved qubit coherence and scalability. Similarly, Intel has ongoing R&D into cryogenic technologies, actively exploring spin-based logic and memory modules compatible with sub-Kelvin operation for quantum and neuromorphic computing applications.
Commercial implementations are still in the early stages, but momentum is building due to the unique advantages of superconducting spintronics—namely, zero-resistance interconnects, ultra-fast switching, and compatibility with cryogenic quantum systems. Organizations such as Toshiba Corporation and National Institute for Materials Science (NIMS) have demonstrated prototype cryogenic spintronic memories and logic gates, with projected scaling to tens of thousands of devices within the next few years.
From a demand perspective, the primary growth drivers include:
- Expansion of quantum computing platforms, which require cryogenic environments and benefit from superconducting spintronic interconnects and memory for scalability and energy efficiency.
- Developments in cryogenic digital electronics for HPC, where superconducting spintronics can slash energy consumption compared to conventional CMOS at low temperatures.
- Emergence of ultra-sensitive magnetic sensors and detectors for scientific instrumentation and medical diagnostics, leveraging the low noise and high sensitivity of spintronic-superconducting hybrids.
Outlook for the period 2025–2030 suggests an annual growth rate in the double digits, as more prototype devices transition to early commercial deployment, particularly in quantum data centers and government-funded research labs. Key constraints include manufacturing scalability, integration with existing cryogenic infrastructure, and the need for robust, reproducible fabrication of hybrid superconducting/spintronics materials.
In summary, as major industry leaders and research institutions accelerate their investment and technical development, the cryogenic superconducting spintronics market is set to evolve from niche research to an enabling technology for the quantum and cryogenic electronics sectors over the next five years.
Technology Evolution: From Lab to Real-World Cryogenic Spintronics
Cryogenic superconducting spintronics—a field at the intersection of superconductivity and spin-based electronics—is poised for significant advances between 2025 and the following few years. Initially rooted in fundamental research, the field has transitioned toward scalable device architectures and integration with existing superconducting platforms and quantum technologies. This evolution is driven by both academic breakthroughs and strategic investments from companies and government initiatives.
By 2025, practical demonstrations of superconducting spintronic devices—such as Josephson junctions incorporating ferromagnetic barriers—are shifting from laboratory environments toward pilot-scale fabrication. Notably, companies specializing in superconducting electronics, such as IBM and MITRE, have invested in cryogenic spintronics as part of their efforts to realize scalable quantum and classical superconducting circuits. Efforts focus on integrating magnetic memory elements (like cryogenic MRAM) and enabling ultra-fast, low-dissipation logic at temperatures compatible with superconducting quantum circuits.
The technology’s progress is partly enabled by advances in thin-film deposition and nanofabrication, refining the interfaces between ferromagnetic and superconducting layers. Organizations like National Institute for Materials Science (NIMS) are developing materials with tailored spin and superconducting properties, supporting reproducible device behavior and scalability. Meanwhile, IBM’s ongoing research explores hybrid architectures that combine superconducting qubits with spintronic elements, aiming for novel memory and logic functionalities that could outperform conventional approaches in both speed and energy efficiency.
Another key trend is the convergence of cryogenic spintronics with the rapidly growing quantum computing sector. As superconducting quantum computers become increasingly viable, the need for cryogenic, non-volatile memory and efficient logic grows. Companies such as Intel are investigating cryogenic-compatible spintronic memory solutions to address data bottlenecks and energy concerns in large-scale quantum systems.
Looking ahead to the next few years, the roadmap includes scaling up device arrays, improving interface stability, and further lowering energy dissipation. Collaborations between government research labs, universities, and industry (such as those coordinated by MITRE and NIMS) are expected to accelerate technology readiness levels. The next milestones involve demonstrating integrated cryogenic spintronic circuits with reproducible performance, targeting applications in superconducting data centers, secure communications, and scalable quantum computing.
While significant engineering challenges remain, the momentum in 2025 and beyond indicates that cryogenic superconducting spintronics will transition from experimental devices to enabling technologies in high-performance computing and quantum infrastructure in the near future.
Superconducting Materials: Innovations, Challenges, and Industry Leaders
Cryogenic superconducting spintronics has emerged as a promising frontier in the quest for ultra-efficient, high-speed computing and quantum information technologies. This field exploits the interplay between superconductivity and spintronic phenomena—leveraging the zero-resistance state of superconductors and the spin degree of freedom in electrons—to realize devices with potential for non-volatile memory, ultra-fast logic, and highly sensitive detectors. As of 2025, several key developments and challenges define the landscape of this sector.
Recent years have witnessed significant progress in the integration of ferromagnetic and superconducting materials at cryogenic temperatures, a necessary step for practical superconducting spintronic devices. Materials such as niobium (Nb), niobium nitride (NbN), and various ferromagnetic alloys are being optimized for interface transparency and low-temperature compatibility. Leading suppliers of high-purity superconducting materials, including American Elements and Kurt J. Lesker Company, are expanding their catalogues to meet specialized demands for thin films and multilayer structures essential for these applications.
On the device side, the most notable advances involve Josephson junctions incorporating ferromagnetic layers, which can exhibit a so-called “π-state” and controllable phase shifts—central elements for superconducting memory and logic. Prototypes of cryogenic memory cells based on these principles are being demonstrated, with companies such as NVE Corporation and research partners focusing on scaling and integration. Meanwhile, Oxford Instruments and Bluefors continue to provide cryogenic measurement systems and dilution refrigerators that enable reliable testing and characterization at millikelvin temperatures.
Challenges persist, particularly in interface engineering to minimize spin scattering and magnetic noise, as well as in scaling fabrication processes from laboratory demonstrations to manufacturable arrays. The need for precise control at the atomic level, especially in hybrid systems combining superconductors with two-dimensional materials or topological insulators, is driving collaborations between materials suppliers, tool manufacturers, and device developers. This collaborative approach is exemplified by partnerships between deposition toolmakers like Plassys and research institutions aiming to commercialize next-generation superconducting spintronic circuits.
Looking ahead to the next few years, the outlook for cryogenic superconducting spintronics is optimistic. Key industry players are ramping up pilot-scale production and device validation, anticipating integration into cryogenic computing platforms and quantum circuits. The demand for ultra-low-power, high-density cryogenic memory and logic solutions is likely to increase, especially as quantum computing and advanced sensor markets expand, positioning superconducting spintronics as a pivotal enabling technology for the next generation of information processing systems.
Quantum Computing Applications: Integrating Spintronics for Next-Gen Performance
Cryogenic superconducting spintronics is emerging as a key enabler for the next generation of quantum computing applications, driven by the need for ultra-low power, high-speed, and highly scalable devices operating at millikelvin temperatures. By leveraging the quantum properties of electron spin along with superconductivity, these hybrid devices promise to overcome some of the critical bottlenecks in current quantum architectures, particularly those based on superconducting qubits.
In 2025, research and industry focus is intensifying around the integration of spintronic elements—such as ferromagnetic Josephson junctions and spin valves—into superconducting circuits. These efforts are motivated by the potential to introduce non-volatility, fast cryogenic memory, and on-chip logic directly compatible with quantum processors. Notably, the introduction of magnetic Josephson junctions as memory elements allows for rapid, energy-efficient cryogenic RAM, which is considered essential for scalable quantum-classical hybrid systems.
Industry leaders such as IBM are actively developing superconducting quantum processors, and recent collaborations with academic and research institutions have explored the incorporation of spintronic memory elements to reduce the overhead required for classical control at cryogenic temperatures. Similarly, Intel has publicly discussed the challenges in scaling quantum systems and has invested in research on cryogenic control electronics, where spintronic-superconducting hybrids could play a pivotal role by enabling denser, more power-efficient interconnects.
Manufacturers of superconducting materials and cryogenic systems, such as Oxford Instruments, are also expanding their product lines to support rapid prototyping and integration of magnetic and superconducting components at sub-Kelvin temperatures. This ecosystem support is expected to accelerate the transition from laboratory research to pilot-scale deployment in quantum computing testbeds over the next few years.
Key technical milestones anticipated by 2027 include the demonstration of multi-bit cryogenic memory arrays with switching energies below 10-19 J/bit and integration of spintronic logic gates compatible with superconducting qubit architectures. These advances are being tracked by international R&D consortia and standards bodies, such as the IEEE, which are beginning to outline interoperability and benchmarking requirements for quantum-classical co-design involving spintronic-superconducting devices.
Looking ahead, the outlook for cryogenic superconducting spintronics is strong, with projected adoption in advanced quantum computing platforms and potential spillover into high-performance classical cryogenic computing for applications in space, defense, and scientific instrumentation. Continued investment from both established players and specialized startups suggests that the field will see rapid technological maturation and initial commercial deployments in the near term.
Data Storage and Memory: Cryogenic Spintronics as a Game Changer
Cryogenic superconducting spintronics is rapidly emerging as a transformative technology for data storage and memory applications, particularly in a landscape increasingly defined by quantum computing and high-performance computing (HPC) demands. In 2025 and the coming years, the integration of spintronic mechanisms with superconducting circuits at cryogenic temperatures is anticipated to deliver unprecedented energy efficiency, speed, and scalability, potentially reshaping the memory hierarchy in both classical and quantum computing environments.
One of the main drivers behind cryogenic spintronics is the need for ultra-low-power, fast, and dense memory solutions that can operate at the cryogenic temperatures required by superconducting quantum processors. Traditional CMOS-based memory technologies present significant power consumption and thermal management challenges at these temperatures, spurring interest in alternatives like superconducting magnetic random-access memory (S-MRAM) and Josephson junction-based memory cells. These devices exploit the spin degree of freedom and dissipationless current flow enabled by superconductivity, drastically reducing energy consumption and providing switching times in the picosecond regime.
Industry leaders such as IBM and Intel are actively engaged in research and development of cryogenic-compatible memory and logic elements, recognizing their importance for scalable quantum computing architectures. In parallel, specialized companies like Seeqc are working to commercialize digital superconducting electronics, including memory technologies that leverage spintronic and superconducting phenomena to support large-scale quantum processors. These efforts are supported by collaborative initiatives with academic and government laboratories worldwide, aiming to overcome current material and device integration challenges.
Recent demonstrations have shown that hybrid devices combining ferromagnetic and superconducting materials can achieve nonvolatile memory effects at temperatures below 1 Kelvin, while maintaining compatibility with superconducting digital logic. This paves the way for multi-megabit cryogenic memory arrays, which are essential for controlling and reading out large numbers of qubits in future quantum computers. Furthermore, the use of spin-polarized supercurrents and spin-triplet proximity effects is being explored to enhance device performance and scalability.
Looking ahead, the next few years are expected to see the first prototype systems that integrate cryogenic superconducting spintronic memory with quantum processors, as well as the broader adoption of these technologies in HPC systems that require ultra-low latency and high bandwidth. Progress in material science, nanofabrication, and low-temperature electronics will be key to moving from proof-of-concept devices to manufacturable products. As the ecosystem develops, partnerships between technology developers like IBM, Intel, and superconducting electronics firms such as Seeqc will likely accelerate innovation and adoption in this field.
Competitive Landscape: Key Players and Strategic Moves (e.g., ibm.com, ieee.org)
The competitive landscape for cryogenic superconducting spintronics is rapidly evolving as leading technology companies and research institutions intensify efforts to commercialize and scale quantum and superconducting electronics. In 2025, much of the sector’s innovation is driven by collaborations between industry leaders, academic researchers, and government-backed research labs, with a focus on advancing device performance, integration, and manufacturability.
Among the most prominent players, IBM continues to spearhead research and development in superconducting quantum circuits, leveraging their expertise in quantum computing hardware. Their work on integrating spintronics at cryogenic temperatures has yielded significant advancements in low-loss interconnects and scalable device architectures, positioning them as a key driver of the field’s direction. Strategic partnerships with universities and national laboratories enable IBM to maintain a leadership position as the demand for more efficient cryogenic electronics grows.
In parallel, Intel is actively investing in cryogenic control technologies, aiming to overcome the scalability bottlenecks of large-scale quantum processors. Their focus on CMOS-based cryogenic controllers and hybrid superconducting-spintronics devices underlines a broader industry trend toward integrating conventional and quantum-compatible electronics for next-generation computing platforms. Recent announcements highlight Intel’s commitment to scaling up quantum and superconducting device manufacturing capabilities to meet anticipated commercial demand in the coming years.
On the materials and device innovation front, companies such as NVE Corporation are notable for their work in spintronics sensors and cryogenic magnetic memory. Their advancements in magnetoresistive materials and spin-valve structures are directly applicable to the cryogenic regime, supporting the development of ultra-low-power memory and logic components. Additionally, Applied Materials plays a strategic role as a key supplier of deposition and characterization equipment tailored for advanced superconducting and spintronic material stacks.
The role of standardization and knowledge exchange is also pivotal, with organizations such as the IEEE facilitating technical standards, conferences, and working groups dedicated to superconducting electronics and spintronics integration. These forums accelerate the adoption of best practices and enable pre-competitive collaboration among stakeholders.
Looking forward, the next few years will likely witness intensified competition for intellectual property, as well as strategic alliances and investments aimed at achieving manufacturable, robust cryogenic spintronic devices. The sector’s trajectory will be shaped by ongoing innovation in materials, device engineering, and scalable cryogenic systems, as major players position themselves for leadership in quantum and high-performance computing hardware markets.
Regulatory, Standardization, and Ecosystem Developments (Refer to ieee.org)
The landscape for cryogenic superconducting spintronics is experiencing pivotal regulatory, standardization, and ecosystem developments as the field transitions from fundamental research to early-stage commercialization. In 2025, efforts are being led primarily by international standards bodies and industry consortia to address the unique requirements of superconducting and spin-based devices operating at cryogenic temperatures.
A central figure in this transition is the IEEE, which has established working groups dedicated to the standardization of superconducting electronics interfaces, device characterization, and measurement protocols. The IEEE P3155 Working Group, for instance, is developing standards for the characterization of superconductor digital electronics, which increasingly include spintronic elements as research progresses. These efforts are intended to facilitate interoperability and reproducibility across different manufacturers and research institutions, a critical step as cryogenic spintronic components begin to enter pilot applications in quantum computing and high-performance computing.
Ecosystem development is also being supported by collaborations between leading technology suppliers and research institutes. For instance, companies such as IBM and Intel are actively engaged in the cryogenic electronics space, integrating superconducting and spintronic elements into their quantum computing roadmaps. Their participation in international roadmapping efforts, such as those coordinated by the International Roadmap for Devices and Systems (IRDS), is helping to align industry priorities and identify pre-competitive challenges in material purity, cryogenic interface standards, and scalability.
On the regulatory front, compliance with cryogenic device safety and environmental regulations is becoming more prominent as larger-scale testbeds are deployed. International bodies such as the International Organization for Standardization (ISO) are working to update and harmonize relevant safety and environmental standards for cryogenic systems, including those incorporating superconducting spintronic devices.
Looking forward to the next few years, the pace of progress is expected to accelerate as more industry players enter the field and as standards mature. The widespread adoption of common measurement and interface protocols will be crucial for fostering a robust supply chain and accelerating technology transfer from research labs to commercial systems, especially as quantum and high-performance computing markets begin to demand scalable cryogenic hardware. Strategic engagement with standards bodies like IEEE and active participation in ecosystem consortia will remain essential for stakeholders seeking to shape the regulatory and interoperability landscape of cryogenic superconducting spintronics.
Investment, Partnerships, and M&A Activity Shaping the Sector
Cryogenic superconducting spintronics is rapidly emerging as a focal point for investment, partnerships, and strategic M&A activity, particularly as quantum computing and next-generation high-performance computing drive demand for ultrafast, energy-efficient electronic devices. In 2025, the sector continues to see notable financing rounds and alliances, especially among companies and institutions at the intersection of quantum hardware, superconducting electronics, and advanced materials.
Key players such as IBM, Intel Corporation, and Google are actively investing in the development of cryogenic and superconducting technologies, recognizing their critical role in quantum processors and cryogenic memory. These investments are often complemented by collaborations with specialized suppliers of cryogenic systems and superconducting materials, including Oxford Instruments and Bluefors, who have expanded their manufacturing capabilities and partnered with quantum computing ventures to deliver tailored cryogenic platforms.
Mergers and acquisitions are also shaping the sector’s landscape. The acquisition of cryogenic component manufacturers or superconducting spintronics startups is being used by established semiconductor and quantum hardware firms as a strategy to vertically integrate and secure critical intellectual property. For example, Nordic Semiconductor and Teledyne Technologies have both signaled intentions to broaden their portfolios into superconducting and spintronic device markets, leveraging acquisitions and strategic investments in early-stage spintronics innovators.
Consortia and public-private partnerships are central to accelerating innovation. The European Quantum Flagship initiative and the U.S. National Quantum Initiative have both facilitated collaborations between universities, national labs, and industry participants such as IBM and Intel Corporation. These alliances focus on scaling cryogenic superconducting spintronic devices for quantum and classical computing applications, pooling resources for fabrication facilities and standardization efforts.
Looking ahead to 2025 and beyond, the sector is poised for further deal activity as demand from quantum computing and high-speed data centers intensifies. The entry of global electronics manufacturers and renewed venture capital interest suggest increasing competition for breakthrough spintronic and superconducting solutions. With strategic alliances and targeted acquisitions, established technology leaders and agile startups alike are positioning themselves to shape the infrastructure underpinning future information technologies.
Future Outlook: Emerging Opportunities and Disruptive Scenarios Through 2030
Cryogenic superconducting spintronics is poised to become a cornerstone technology in next-generation computing and sensing, driven by the unique advantages of combining superconductivity with spin-based information processing. As of 2025, the field is rapidly advancing, with substantial investments and collaborations among leading industry players and research institutes focused on quantum computing, ultra-fast logic devices, and energy-efficient memory architectures.
Key industry participants such as IBM and Intel have intensified research efforts into cryogenic electronics, targeting scalable quantum processors and the integration of superconducting spintronics for improved coherence times and lower energy dissipation. IBM, for example, is actively exploring superconducting quantum circuits, which benefit from cryogenic spintronic interfaces to achieve better control over quantum states and reduced thermal noise.
In Europe, Infineon Technologies and collaborative research consortia are investigating the deployment of superconducting spintronic elements for cryogenic control electronics, essential for quantum computing scalability. Beyond quantum applications, superconducting spintronics are envisioned to revolutionize cryogenic memory and logic—areas critical for data centers and high-performance computing—by enabling ultra-low power consumption and unprecedented data retention at cryogenic temperatures.
Emerging opportunities through 2030 include the commercialization of non-volatile cryogenic MRAM (magnetoresistive random-access memory) for quantum computers, with initiatives from companies such as Toshiba and IBM, both of which have demonstrated prototype devices leveraging superconducting-ferromagnetic junctions. These devices promise to overcome existing limitations of conventional semiconductor memories when operated at millikelvin temperatures.
Disruptive scenarios anticipated in the next five years involve the integration of cryogenic spintronic interconnects and logic gates in quantum and classical hybrid systems. Such developments could lead to a paradigm shift in how data is processed and transmitted in large-scale quantum computers and AI accelerators, enabling new architectures that are not feasible with current CMOS technology. Industry leaders like Intel and IBM are expected to pilot early-stage hybrid systems by 2027–2028.
Looking forward, the maturation of cryogenic superconducting spintronics will depend on scalable fabrication methods, advances in cryo-compatible materials, and the establishment of robust supply chains for superconducting and spintronic components. As these technical hurdles are addressed, the sector is expected to witness rapid market expansion, particularly in quantum computing, high-density memory, and advanced cryogenic sensing applications.