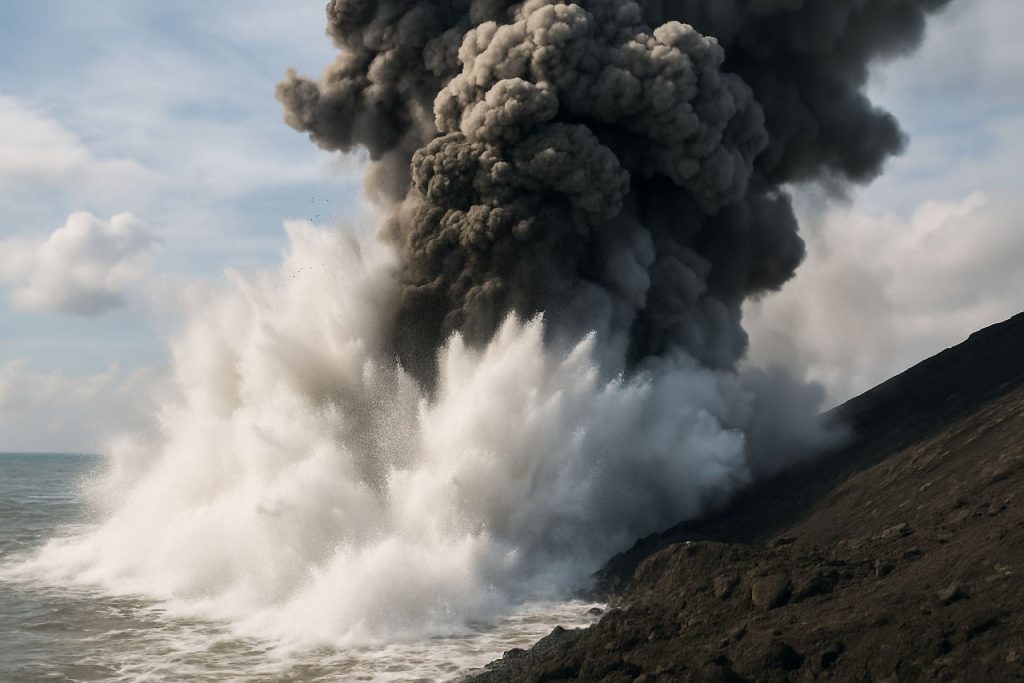
Unleashing Nature’s Steam-Fueled Fury: A Comprehensive Exploration of Phreatomagmatic Eruptions and Their Catastrophic Impact. Discover How Water and Magma Combine to Create Earth’s Most Violent Volcanic Events.
- Introduction to Phreatomagmatic Eruptions
- Physical and Chemical Mechanisms Behind the Explosions
- Historical Case Studies: Notable Phreatomagmatic Events
- Distinguishing Features: Phreatomagmatic vs. Other Eruption Types
- Deposits and Geological Signatures
- Hazards and Environmental Impacts
- Monitoring and Predicting Phreatomagmatic Activity
- Role in Shaping Volcanic Landscapes
- Implications for Volcanic Hazard Mitigation
- Future Research Directions and Unanswered Questions
- Sources & References
Introduction to Phreatomagmatic Eruptions
Phreatomagmatic eruptions are a distinct type of volcanic activity that occurs when magma interacts explosively with external water sources, such as groundwater, lakes, or seawater. This interaction leads to rapid cooling and fragmentation of magma, resulting in highly energetic explosions that differ significantly from purely magmatic or phreatic eruptions. The term “phreatomagmatic” is derived from the Greek word “phrear,” meaning well or spring, and “magmatic,” referring to molten rock. These eruptions are characterized by the violent expansion of steam generated as water comes into contact with hot magma, causing the magma to shatter into fine ash and volcanic debris.
The dynamics of phreatomagmatic eruptions are complex, involving both thermal and mechanical processes. When water infiltrates a magma chamber or comes into contact with erupting lava, it is rapidly heated and converted to steam. The sudden increase in pressure from steam formation can fragment both the magma and the surrounding rock, producing ash-rich eruption columns and base surges. These eruptions often generate distinctive deposits, such as tuff rings and maars, which are low-relief volcanic craters formed by explosive interactions between magma and water.
Phreatomagmatic eruptions are significant not only for their explosive power but also for their potential hazards. The fine ash produced can travel long distances, impacting air quality and aviation safety. Additionally, the rapid expansion of steam can generate pyroclastic surges—fast-moving, ground-hugging clouds of hot gas and volcanic particles—that pose serious risks to nearby populations and infrastructure. Understanding the mechanisms and precursors of phreatomagmatic eruptions is therefore a key focus for volcanological research and hazard mitigation.
Globally, several prominent volcanoes have exhibited phreatomagmatic activity, including Surtsey in Iceland and Taal in the Philippines. Organizations such as the United States Geological Survey and the British Geological Survey play crucial roles in monitoring volcanic activity, studying eruption mechanisms, and providing guidance on risk reduction. Their research contributes to a deeper understanding of phreatomagmatic processes and supports the development of early warning systems for communities living near active volcanoes.
Physical and Chemical Mechanisms Behind the Explosions
Phreatomagmatic eruptions are a distinct class of volcanic activity characterized by the explosive interaction between magma and external water sources, such as groundwater, lakes, or seawater. The physical and chemical mechanisms driving these eruptions are complex, involving rapid heat transfer, phase changes, and fragmentation processes that distinguish them from purely magmatic or phreatic eruptions.
The primary physical mechanism behind phreatomagmatic explosions is the rapid transfer of heat from ascending magma to water. When magma comes into contact with water, the intense temperature difference causes the water to flash into steam almost instantaneously. This phase change is accompanied by a dramatic increase in volume—steam occupies over 1,700 times the volume of liquid water at atmospheric pressure. The sudden expansion generates powerful shock waves, fragmenting both the magma and the surrounding country rock. This process, known as “fuel-coolant interaction,” is highly efficient at producing fine ash and fragmented material, which are characteristic of phreatomagmatic deposits.
Chemically, the interaction between magma and water can also induce significant changes. The rapid quenching of magma by water leads to the formation of volcanic glass and fine ash particles. Additionally, the presence of water can promote the leaching of soluble elements from the magma, altering the composition of the erupted material. The incorporation of external water also increases the explosivity of the eruption by enhancing the fragmentation efficiency and by generating large volumes of steam-driven gas. This is in contrast to magmatic eruptions, where the primary driving force is the exsolution of volatiles (such as water vapor and carbon dioxide) originally dissolved in the magma itself.
The efficiency of fragmentation in phreatomagmatic eruptions is further influenced by the ratio of water to magma, the permeability of the surrounding rocks, and the confining pressure. If the water supply is abundant and the interaction is sustained, the eruption can produce towering columns of ash and steam, as well as base surges—ground-hugging, turbulent clouds of ash and gas. These phenomena are well-documented in classic phreatomagmatic eruptions such as those at Surtsey (Iceland) and Taal (Philippines).
Understanding the physical and chemical mechanisms of phreatomagmatic eruptions is crucial for hazard assessment and risk mitigation, as these eruptions can be highly explosive and unpredictable. Organizations such as the United States Geological Survey and the Global Volcanism Program of the Smithsonian Institution conduct ongoing research and monitoring to better characterize these processes and their implications for communities living near active volcanoes.
Historical Case Studies: Notable Phreatomagmatic Events
Phreatomagmatic eruptions, characterized by the explosive interaction between magma and external water sources, have produced some of the most dramatic and scientifically significant volcanic events in history. These eruptions are distinguished by their violent fragmentation of magma, often resulting in widespread ash dispersal, base surges, and the formation of unique volcanic landforms. Several historical case studies illustrate the diverse impacts and scientific insights gained from notable phreatomagmatic events.
One of the most studied phreatomagmatic eruptions is the 1883 eruption of Krakatoa in Indonesia. This catastrophic event was triggered by the interaction of seawater with rising magma, leading to a series of massive explosions. The eruption generated powerful tsunamis and ejected vast quantities of ash and pumice into the atmosphere, causing global climatic effects. The event remains a benchmark for understanding the hazards associated with phreatomagmatic activity and the potential for far-reaching environmental consequences (United States Geological Survey).
Another significant example is the 1963 eruption of Surtsey, a volcanic island off the southern coast of Iceland. The eruption began underwater and transitioned to a phreatomagmatic phase as the volcano breached the ocean surface. The Surtsey eruption provided a rare opportunity for scientists to observe the formation of new land and the processes of primary succession in a pristine environment. The event also contributed to the development of the term “Surtseyan eruption,” now used to describe similar shallow-water phreatomagmatic activity (Icelandic Meteorological Office).
The 1977 eruption of Ukinrek Maars in Alaska offers another instructive case. This event involved the explosive interaction of magma with groundwater, resulting in the rapid formation of two maars—broad, low-relief volcanic craters. The Ukinrek Maars eruption was notable for its well-documented sequence and the preservation of eruption deposits, providing valuable data on the mechanics of phreatomagmatic explosions and maar formation (Alaska Volcano Observatory).
These historical case studies underscore the importance of phreatomagmatic eruptions in shaping volcanic landscapes and influencing hazard assessments. They also highlight the role of scientific organizations, such as the United States Geological Survey, Icelandic Meteorological Office, and Alaska Volcano Observatory, in monitoring, researching, and disseminating knowledge about these complex volcanic phenomena.
Distinguishing Features: Phreatomagmatic vs. Other Eruption Types
Phreatomagmatic eruptions are a distinct class of volcanic activity characterized by the explosive interaction between magma and external water sources, such as groundwater, lakes, or seawater. This interaction sets them apart from other eruption types, notably magmatic and phreatic eruptions, through several key features.
The primary distinguishing feature of phreatomagmatic eruptions is the direct contact between hot magma and water, which leads to rapid vaporization and a dramatic increase in pressure. This process, known as “fuel-coolant interaction,” results in highly explosive fragmentation of both magma and surrounding rock. The resulting eruptions typically produce fine-grained ash, blocky tephra, and base surges—ground-hugging, turbulent clouds of ash and gas that radiate outward from the vent. These base surges are a hallmark of phreatomagmatic activity and are rarely observed in purely magmatic eruptions.
In contrast, magmatic eruptions are driven primarily by the exsolution of volatiles (gases) dissolved within the magma itself. These eruptions can range from effusive lava flows to highly explosive events, but the fragmentation is generally less efficient than in phreatomagmatic eruptions, resulting in coarser tephra and a lack of the characteristic base surges. The products of magmatic eruptions often include pumice and scoria, which are less common in phreatomagmatic deposits.
Phreatic eruptions, on the other hand, involve the explosive expansion of steam generated by the heating of groundwater or surface water, but without direct involvement of fresh magma. These eruptions typically eject pre-existing rock fragments and steam, with little to no juvenile (newly formed) volcanic material. The absence of new magma distinguishes phreatic from phreatomagmatic eruptions, which always involve the fragmentation of fresh magma.
Another distinguishing aspect of phreatomagmatic eruptions is their depositional features. The ash and tephra produced are often highly fragmented, angular, and fine-grained, reflecting the violent nature of the magma-water interaction. Deposits may show evidence of wet emplacement, such as accretionary lapilli—small, rounded ash aggregates formed in moist eruption columns. These features are less common in magmatic or phreatic deposits.
Globally, phreatomagmatic eruptions have been responsible for the formation of unique volcanic landforms, such as maars (broad, low-relief craters) and tuff rings. Organizations like the United States Geological Survey and the British Geological Survey provide detailed documentation and monitoring of such eruptions, contributing to the understanding of their hazards and distinguishing characteristics.
Deposits and Geological Signatures
Phreatomagmatic eruptions are characterized by the explosive interaction between magma and external water sources, such as groundwater, lakes, or seawater. This interaction produces distinctive deposits and geological signatures that are critical for interpreting past volcanic activity and assessing volcanic hazards. The deposits from phreatomagmatic eruptions are typically more fragmented and finer-grained than those from purely magmatic eruptions, reflecting the intense fragmentation caused by rapid steam expansion.
One of the hallmark deposits of phreatomagmatic activity is tuff, particularly base surge and ash surge deposits. These are often well-bedded, laterally extensive, and display cross-bedding or dune-like structures, indicating emplacement by ground-hugging, turbulent surges. The fine-grained nature of these deposits, with abundant ash-sized particles and accretionary lapilli (rounded ash aggregates formed in moist eruption columns), distinguishes them from the coarser, more massive deposits of magmatic eruptions. The presence of palagonitized glass shards—volcanic glass altered by interaction with water—is another diagnostic feature of phreatomagmatic deposits.
Geologically, phreatomagmatic eruptions often produce maar craters and tuff rings. Maars are broad, low-relief craters formed by explosive excavation of the substrate, typically surrounded by rings of bedded tuff. Tuff rings are similar but tend to be larger and have more pronounced rims. These landforms are key indicators of past phreatomagmatic activity and are widely studied in volcanic fields worldwide. The United States Geological Survey (USGS), a leading authority in volcanology, has documented numerous examples of such features in regions like the western United States and Alaska.
Microscopically, phreatomagmatic deposits often contain a high proportion of angular, blocky glass shards and lithic fragments, reflecting the mechanical fragmentation of both magma and country rock. The presence of hydrothermal alteration minerals—such as clays and zeolites—within these deposits further attests to the role of water in their formation. These mineralogical and textural signatures are used by geologists to distinguish phreatomagmatic deposits from those of other eruption types.
In summary, the deposits and geological signatures of phreatomagmatic eruptions—fine-grained, well-bedded tuffs, accretionary lapilli, palagonitized glass, maar craters, and tuff rings—provide essential evidence for reconstructing eruption histories and understanding the hazards associated with water-magma interactions. Organizations such as the British Geological Survey and the United States Geological Survey continue to study these features to improve volcanic risk assessment and land use planning in vulnerable regions.
Hazards and Environmental Impacts
Phreatomagmatic eruptions are a distinct type of volcanic activity that occurs when magma interacts explosively with external water sources, such as groundwater, lakes, or seawater. This interaction leads to rapid steam generation, fragmentation of magma, and violent ejection of ash, gas, and rock fragments. The hazards and environmental impacts associated with phreatomagmatic eruptions are significant and multifaceted, affecting both local and global environments.
One of the primary hazards of phreatomagmatic eruptions is the production of fine-grained volcanic ash. The explosive interaction between water and magma shatters the magma into tiny particles, which can be dispersed over vast areas by wind. Ash fallout can disrupt air traffic, damage infrastructure, contaminate water supplies, and pose respiratory health risks to humans and animals. The fine ash is also capable of causing long-term soil and water contamination, impacting agriculture and ecosystems. The United States Geological Survey (USGS), a leading authority in volcanology, has documented numerous cases where phreatomagmatic ash clouds have led to widespread disruption and environmental damage.
Another significant hazard is the generation of base surges and pyroclastic density currents. These ground-hugging, fast-moving clouds of hot gas, ash, and volcanic debris can travel several kilometers from the eruption site, destroying everything in their path. Such surges are particularly dangerous due to their speed and high temperatures, posing a severe threat to life and property. The British Geological Survey (BGS), a prominent geological research organization, highlights that base surges are characteristic of phreatomagmatic eruptions and have been responsible for catastrophic impacts in historical events, such as the 1883 Krakatoa eruption.
Phreatomagmatic eruptions can also trigger secondary hazards, including tsunamis when eruptions occur in or near bodies of water. The sudden displacement of water by explosive activity or collapse of volcanic material can generate large waves, threatening coastal communities. Additionally, the rapid cooling and fragmentation of magma can produce large volumes of volcanic glass shards, which are particularly abrasive and hazardous to both mechanical systems and biological tissues.
Environmental impacts extend beyond immediate hazards. The deposition of ash and tephra can alter landscapes, disrupt ecosystems, and affect climate by injecting fine particles into the atmosphere, potentially influencing weather patterns and reducing sunlight. Organizations such as the United Nations Environment Programme (UNEP) monitor and assess the broader environmental consequences of volcanic eruptions, including those of the phreatomagmatic type, to inform risk mitigation and environmental management strategies.
Monitoring and Predicting Phreatomagmatic Activity
Monitoring and predicting phreatomagmatic eruptions—volcanic events driven by the explosive interaction of magma and water—pose unique challenges due to their sudden onset and complex precursors. These eruptions can generate highly hazardous phenomena, including base surges, ash plumes, and ballistic projectiles, making early detection and accurate forecasting critical for risk mitigation.
Modern volcano monitoring systems employ a multi-parameter approach to detect signs of impending phreatomagmatic activity. Seismic monitoring is fundamental, as the movement of magma and the interaction with groundwater often produce distinctive seismic signals. Networks of seismometers, operated by organizations such as the United States Geological Survey (USGS) and the British Geological Survey (BGS), continuously record ground vibrations to identify volcanic tremor, low-frequency events, and sudden increases in seismicity that may precede eruptions.
In addition to seismic data, ground deformation is closely monitored using GPS, tiltmeters, and InSAR (Interferometric Synthetic Aperture Radar) technology. Swelling or subsidence of the volcano’s surface can indicate magma intrusion or changes in hydrothermal systems, both of which are relevant to phreatomagmatic processes. The Global Volcanism Program of the Smithsonian Institution compiles and analyzes such geodetic data from volcanoes worldwide.
Hydrogeological monitoring is particularly important for phreatomagmatic eruptions. Changes in groundwater levels, temperature, and chemistry can signal increased interaction between magma and water. Volcanological observatories, such as those coordinated by the International Volcanic Health Hazard Network (IVHHN), often monitor springs, fumaroles, and crater lakes for anomalies that may precede explosive events.
Gas emissions are another key indicator. Sudden increases in steam, sulfur dioxide, or carbon dioxide emissions can reflect the heating of groundwater or the opening of new pathways for gas escape. Remote sensing and direct sampling are used to track these changes, with data shared among international networks like the World Organization of Volcano Observatories (WOVO).
Despite advances in monitoring, predicting the exact timing and magnitude of phreatomagmatic eruptions remains difficult. These eruptions can occur with little warning, especially when external water sources are rapidly introduced to shallow magma. Therefore, continuous data integration, real-time analysis, and robust communication between scientific agencies and local authorities are essential for effective hazard assessment and public safety.
Role in Shaping Volcanic Landscapes
Phreatomagmatic eruptions play a significant role in shaping volcanic landscapes, often producing distinctive landforms and altering existing topography through their unique eruptive mechanisms. These eruptions occur when magma interacts explosively with external water sources, such as groundwater, lakes, or seawater. The rapid conversion of water to steam leads to violent fragmentation of magma and surrounding rock, resulting in powerful explosions that differ markedly from purely magmatic eruptions.
One of the most characteristic landforms produced by phreatomagmatic activity is the maar, a broad, low-relief volcanic crater typically filled with water to form a lake. Maars are created when explosive interactions between magma and groundwater excavate a crater below the pre-eruption ground level, ejecting fragmented material and leaving a depression. These features are widespread in volcanic fields around the world and serve as clear evidence of past phreatomagmatic events. Another common landform is the tuff ring, a circular or oval ridge composed of consolidated volcanic ash and debris deposited around the eruption site. Tuff rings are generally larger and broader than cinder cones, reflecting the wide dispersal of fine-grained material by phreatomagmatic explosions.
Phreatomagmatic eruptions also contribute to the formation of tuff cones, which are steeper and higher than tuff rings due to the accumulation of wet, cohesive ash that resists erosion. These cones often display well-bedded layers of ash and fragmented rock, indicating repeated explosive interactions with water. The deposits from phreatomagmatic eruptions are typically fine-grained and well-sorted, reflecting the efficiency of steam-driven fragmentation and the dispersal of material by base surges—ground-hugging, turbulent clouds of ash and gas.
Beyond creating new landforms, phreatomagmatic eruptions can dramatically modify existing landscapes. The explosive excavation of craters can disrupt pre-existing volcanic edifices, while the widespread deposition of ash and tuff can blanket large areas, altering drainage patterns and soil characteristics. In coastal or lacustrine settings, phreatomagmatic activity can reshape shorelines and create new islands or peninsulas.
The study of phreatomagmatic landforms provides valuable insights into past hydrological and volcanic conditions, helping volcanologists reconstruct eruption histories and assess hazards. Organizations such as the United States Geological Survey and the British Geological Survey conduct extensive research on these processes, contributing to our understanding of how phreatomagmatic eruptions shape the dynamic volcanic landscapes of our planet.
Implications for Volcanic Hazard Mitigation
Phreatomagmatic eruptions, which occur when magma interacts explosively with external water sources such as groundwater, lakes, or the ocean, present unique challenges for volcanic hazard mitigation. These eruptions are characterized by their high explosivity, fine ash production, and the potential for widespread dispersal of volcanic material. Understanding the implications of phreatomagmatic activity is essential for developing effective hazard mitigation strategies, particularly in regions where water and magma are likely to interact.
One of the primary hazards associated with phreatomagmatic eruptions is the generation of fine-grained ash, which can be transported over large distances by wind. This ash poses significant risks to human health, infrastructure, and aviation. Fine ash can cause respiratory problems, contaminate water supplies, and disrupt transportation networks. The United States Geological Survey (USGS), a leading authority in volcano monitoring and hazard assessment, emphasizes the importance of ash fall forecasting and public communication to minimize these impacts.
Another critical implication is the potential for sudden and violent explosions, which can produce base surges, ballistic projectiles, and pyroclastic density currents. These phenomena can devastate areas close to the eruption site and are difficult to predict due to the complex interplay between magma and water. The British Geological Survey (BGS), which conducts research and monitoring of volcanic hazards, highlights the need for detailed geological mapping and real-time monitoring of groundwater and surface water systems near active volcanoes to anticipate phreatomagmatic activity.
Effective hazard mitigation for phreatomagmatic eruptions requires a multidisciplinary approach. This includes:
- Comprehensive monitoring of volcanic, hydrological, and meteorological conditions to detect early signs of magma-water interaction.
- Development of robust early warning systems and clear communication protocols to inform at-risk populations.
- Land-use planning that restricts development in high-risk zones, particularly around lakes, coastal areas, and regions with shallow groundwater.
- Public education campaigns to raise awareness of the specific dangers posed by phreatomagmatic eruptions and appropriate response actions.
International organizations such as the United Nations Office for Disaster Risk Reduction (UNDRR) also play a vital role by promoting global best practices in disaster risk management and supporting capacity-building initiatives in vulnerable regions. By integrating scientific research, technological advances, and community engagement, authorities can better anticipate, prepare for, and respond to the unique hazards posed by phreatomagmatic eruptions.
Future Research Directions and Unanswered Questions
Phreatomagmatic eruptions, characterized by the explosive interaction between magma and external water sources, remain a complex and partially understood phenomenon within volcanology. Despite significant advances in observational techniques and experimental petrology, several key questions persist, guiding future research directions in this field.
One major area for future investigation is the precise dynamics of magma-water interaction at varying depths and environmental conditions. The efficiency of fragmentation, the role of water-to-magma ratios, and the influence of confining pressure on eruption style are not yet fully quantified. High-resolution geophysical monitoring and laboratory simulations are needed to better constrain these parameters and improve predictive models.
Another critical research direction involves the identification and monitoring of hydrogeological systems surrounding active volcanoes. Understanding the spatial distribution, recharge rates, and connectivity of aquifers or surface water bodies is essential for assessing the likelihood and potential intensity of phreatomagmatic activity. Integrating hydrological data with volcanic monitoring networks could enhance early warning capabilities and risk assessments.
The generation and dispersal of volcanic ash during phreatomagmatic eruptions also present unresolved challenges. The fine-grained nature of phreatomagmatic ash has significant implications for aviation safety, public health, and climate, yet the mechanisms controlling ash particle size, morphology, and aggregation remain incompletely understood. Future research should focus on real-time ash sampling during eruptions and advanced analytical techniques to characterize ash properties.
Additionally, the long-term environmental and ecological impacts of phreatomagmatic eruptions are not fully documented. Studies on the effects of ash deposition on soil chemistry, water quality, and ecosystem recovery are needed, particularly in regions with frequent or large-scale phreatomagmatic events.
International organizations such as the United States Geological Survey and the British Geological Survey play pivotal roles in advancing research through global monitoring networks, data sharing, and collaborative projects. However, there is a recognized need for more interdisciplinary and cross-institutional efforts, especially in under-monitored volcanic regions.
In summary, future research on phreatomagmatic eruptions should prioritize: (1) detailed studies of magma-water interaction dynamics; (2) improved hydrogeological mapping and monitoring; (3) comprehensive analysis of ash generation and dispersal; and (4) assessment of long-term environmental impacts. Addressing these unanswered questions will enhance eruption forecasting, hazard mitigation, and our fundamental understanding of explosive volcanic processes.
Sources & References
- British Geological Survey
- Icelandic Meteorological Office
- Alaska Volcano Observatory
- United Nations Environment Programme
- British Geological Survey
- International Volcanic Health Hazard Network