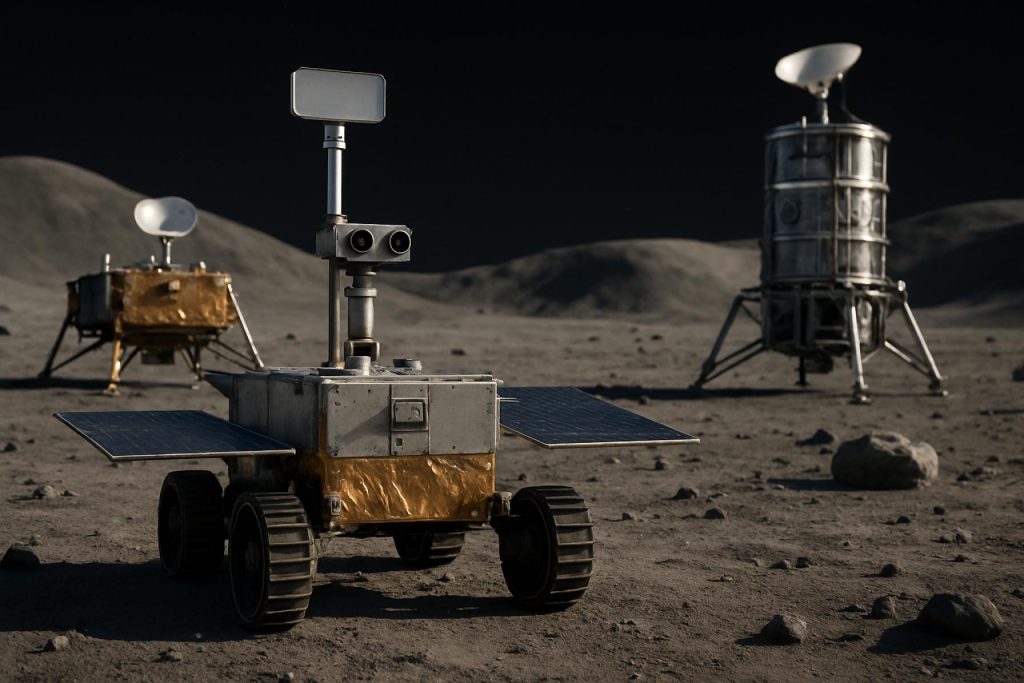
The 2025 Robotic Space Hardware Revolution: How Rovers, Landers, and Probes Are Redefining Planetary Exploration. Discover the Technologies and Market Forces Shaping the Next Five Years.
- Executive Summary: 2025 Market Snapshot & Key Trends
- Market Size & Forecast (2025–2030): Growth Drivers and 18% CAGR Analysis
- Major Players: OEMs, Suppliers, and New Entrants (e.g., nasa.gov, esa.int, isro.gov.in, jaxa.jp, spacex.com)
- Technology Innovations: Mobility, Autonomy, and AI in Rovers, Landers, and Probes
- Materials & Manufacturing: Lightweight Alloys, Composites, and Additive Manufacturing
- Mission Pipeline: Upcoming Lunar, Martian, and Deep Space Deployments
- Supply Chain & Integration: Challenges and Opportunities
- Regulatory & Standards Landscape: International Collaboration and Compliance (e.g., ieee.org, asme.org)
- Investment & Funding Trends: Government, Commercial, and Venture Capital
- Future Outlook: Emerging Markets, Next-Gen Concepts, and Long-Term Impact
- Sources & References
Executive Summary: 2025 Market Snapshot & Key Trends
The market for robotic space exploration hardware—including rovers, landers, and probes—is poised for significant activity and innovation in 2025, driven by both governmental and commercial initiatives. The sector is characterized by a surge in mission launches, technological advancements, and the entry of new players, reflecting a robust outlook for the near future.
In 2025, several high-profile missions are scheduled to deploy advanced robotic hardware. NASA continues to lead with its Artemis program, which, while focused on crewed lunar exploration, also incorporates robotic landers and rovers for surface reconnaissance and logistics. The agency’s VIPER rover, designed to search for water ice at the lunar south pole, is slated for launch in late 2024 or early 2025, marking a milestone in resource prospecting on the Moon. Meanwhile, European Space Agency (ESA) is advancing its ExoMars Rosalind Franklin rover, with a revised launch window targeting 2028, but ongoing hardware development and testing will remain a key market driver through 2025.
Commercial entities are increasingly shaping the landscape. Astrobotic Technology and Intuitive Machines are both contracted under NASA’s Commercial Lunar Payload Services (CLPS) program to deliver landers and small rovers to the lunar surface. Their Peregrine and Nova-C landers, respectively, are expected to demonstrate new capabilities in payload delivery and surface operations, with launches anticipated in 2024–2025. ispace, a Japanese lunar exploration company, is also preparing for its next Hakuto-R missions, aiming to establish a commercial presence on the Moon and support international payloads.
On the planetary front, Japan Aerospace Exploration Agency (JAXA) is advancing its Martian Moons eXploration (MMX) mission, with a lander and rover targeting Phobos in 2026, and hardware development ramping up in 2025. CNES (the French space agency) and DLR (German Aerospace Center) are key partners in European and international rover and lander projects, contributing specialized subsystems and scientific payloads.
Key trends shaping the 2025 market include miniaturization, modularity, and increased autonomy of robotic systems, enabling more cost-effective and flexible missions. The proliferation of commercial lunar landers and the maturation of international partnerships are expected to expand the addressable market for hardware suppliers and subsystem manufacturers. As lunar and planetary exploration intensifies, the demand for robust, adaptable robotic platforms will remain strong, positioning the sector for continued growth and technological evolution through the remainder of the decade.
Market Size & Forecast (2025–2030): Growth Drivers and 18% CAGR Analysis
The global market for robotic space exploration hardware—including rovers, landers, and probes—is poised for robust expansion between 2025 and 2030, with an estimated compound annual growth rate (CAGR) of approximately 18%. This growth is driven by a confluence of factors: increased governmental and commercial investments in planetary science, the emergence of new lunar and Martian exploration programs, and the maturation of enabling technologies such as autonomous navigation, miniaturized sensors, and advanced materials.
Key drivers include the resurgence of lunar exploration, exemplified by NASA’s Artemis program, which is deploying a series of robotic landers and rovers to the Moon’s surface as precursors to human missions. NASA’s Commercial Lunar Payload Services (CLPS) initiative is contracting private sector partners—including NASA and companies like Intuitive Machines and Astrobotic Technology—to deliver scientific payloads and technology demonstrations via robotic landers. These missions, scheduled throughout the latter half of the 2020s, are expected to catalyze demand for advanced lander and rover platforms.
Mars exploration remains a significant market segment, with ongoing and planned missions from agencies such as European Space Agency (ESA), Japan Aerospace Exploration Agency (JAXA), and Indian Space Research Organisation (ISRO). The ESA’s Rosalind Franklin rover, for example, is slated for launch in the coming years, while ISRO is preparing follow-up missions to its successful Chandrayaan and Mars Orbiter programs. These initiatives are expected to drive procurement of sophisticated robotic hardware, including mobility systems, scientific instruments, and autonomous control units.
Commercial players are increasingly active, with companies such as Maxar Technologies supplying robotic arms and spacecraft components for both governmental and private missions. The proliferation of small satellite and CubeSat-based probes, enabled by miniaturization and cost reductions, is further expanding the market, allowing new entrants to participate in deep space exploration.
Looking ahead, the market outlook is buoyed by the anticipated ramp-up of lunar resource prospecting, in-situ resource utilization (ISRU) demonstrations, and the establishment of semi-permanent robotic outposts. The convergence of public-private partnerships, international collaboration, and technological innovation is expected to sustain double-digit growth rates through 2030, with the Asia-Pacific and North American regions leading in both demand and technological capability.
Major Players: OEMs, Suppliers, and New Entrants (e.g., nasa.gov, esa.int, isro.gov.in, jaxa.jp, spacex.com)
The landscape of robotic space exploration hardware in 2025 is defined by a dynamic mix of established agencies, private sector OEMs, and emerging entrants, all contributing to the development and deployment of rovers, landers, and probes. These players are driving innovation in mission architectures, autonomy, and hardware resilience, with a focus on lunar, Martian, and deep space exploration.
- NASA: As a global leader, NASA continues to spearhead major robotic missions. The Perseverance rover, operational on Mars since 2021, is collecting samples for eventual return. NASA’s VIPER lunar rover, scheduled for launch in late 2024, will prospect for water ice at the Moon’s south pole, supporting Artemis program objectives. NASA also collaborates with commercial partners through the Commercial Lunar Payload Services (CLPS) initiative, enabling private landers and payloads to reach the lunar surface.
- ESA: The European Space Agency (ESA) is advancing the Rosalind Franklin ExoMars rover, with a revised launch window targeting 2028 after delays. ESA is also a key partner in the Mars Sample Return campaign, contributing the Sample Transfer Arm and Earth Return Orbiter. Additionally, ESA supports lunar exploration through the European Large Logistics Lander (EL3) concept, aiming for cargo and science delivery to the Moon later this decade.
- ISRO: The Indian Space Research Organisation (ISRO) is building on the success of Chandrayaan-3, which deployed the Pragyan rover on the Moon in 2023. ISRO is planning Chandrayaan-4, a lunar sample return mission, and is developing new lander and rover technologies for future lunar and interplanetary missions.
- JAXA: Japan Aerospace Exploration Agency (JAXA) achieved a milestone with the SLIM lunar lander in 2024, demonstrating pinpoint landing technology. JAXA is also collaborating with international partners on the Martian Moons eXploration (MMX) mission, set to launch in 2026, which will deploy a small rover to Phobos.
- SpaceX: SpaceX is emerging as a key commercial supplier, providing launch services for robotic missions and developing the Starship vehicle, which is expected to deliver large payloads—including landers and rovers—to the Moon and Mars. SpaceX’s involvement in NASA’s Artemis program as a lunar lander provider further cements its role in the sector.
- New Entrants and Suppliers: The sector is witnessing increased participation from private companies such as Astrobotic, Intuitive Machines, and ispace, each developing lunar landers under NASA’s CLPS and other programs. These firms are advancing modular, commercial hardware platforms for science and resource prospecting missions.
Looking ahead, the next few years will see a surge in robotic hardware launches, with a focus on lunar surface operations, Mars sample return, and technology demonstrations for future human exploration. The interplay between established agencies and agile private firms is expected to accelerate innovation and expand the global footprint of robotic exploration.
Technology Innovations: Mobility, Autonomy, and AI in Rovers, Landers, and Probes
The period around 2025 marks a significant leap in the technological sophistication of robotic space exploration hardware, particularly in the domains of mobility, autonomy, and artificial intelligence (AI) for rovers, landers, and probes. These advancements are being driven by both governmental space agencies and private sector innovators, with a focus on enabling more complex, longer-duration, and farther-reaching missions across the solar system.
Mobility systems are undergoing rapid evolution, with new designs aimed at traversing diverse and challenging terrains. For example, the upcoming NASA VIPER lunar rover, scheduled for launch in late 2024, features independently driven wheels and advanced suspension to navigate the Moon’s south pole, where it will search for water ice. Similarly, the European Space Agency’s (ESA) Rosalind Franklin rover, part of the ExoMars program, is equipped with a unique drill capable of reaching two meters below the Martian surface, and a sophisticated wheel-walking mechanism to overcome soft soils and obstacles.
Autonomy is a critical area of innovation, as communication delays with Earth necessitate that robotic explorers make real-time decisions. The Mars 2020 Perseverance rover, developed by NASA and operational since 2021, set a new standard with its onboard Terrain-Relative Navigation system, allowing it to autonomously select safe landing sites and traverse hazardous landscapes. Building on this, future missions are integrating more advanced AI-driven navigation and hazard avoidance, with JAXA (Japan Aerospace Exploration Agency) and ISRO (Indian Space Research Organisation) both planning lunar and planetary landers that leverage machine learning for surface operations.
AI is also transforming scientific operations and mission planning. Rovers and probes are increasingly equipped with onboard data analysis capabilities, enabling them to prioritize scientific targets, adapt sampling strategies, and even detect novel phenomena without waiting for instructions from Earth. For instance, NASA is developing AI-based software for its upcoming Mars Sample Return campaign, allowing hardware to autonomously identify and cache the most promising samples. Meanwhile, private companies such as Astrobotic Technology and Intuitive Machines are integrating autonomous navigation and AI-driven payload management into their commercial lunar landers, supporting NASA’s Artemis program and international partners.
Looking ahead, the convergence of advanced mobility, autonomy, and AI is expected to enable robotic explorers to operate in previously inaccessible environments, such as permanently shadowed lunar craters, Martian caves, and the icy surfaces of outer solar system moons. These innovations will not only expand the scientific return of each mission but also lay the groundwork for future human-robotic collaboration in space exploration.
Materials & Manufacturing: Lightweight Alloys, Composites, and Additive Manufacturing
The development of robotic space exploration hardware—encompassing rovers, landers, and probes—relies heavily on advanced materials and manufacturing techniques to meet the stringent demands of extraterrestrial environments. As of 2025 and looking ahead, the sector is witnessing significant advancements in lightweight alloys, composites, and additive manufacturing, all of which are critical for enhancing mission payload efficiency, durability, and adaptability.
Lightweight alloys, particularly those based on aluminum, titanium, and magnesium, remain foundational in the construction of structural frames and mobility systems for robotic explorers. These materials offer high strength-to-weight ratios, essential for minimizing launch mass while ensuring resilience against the mechanical stresses of launch, landing, and surface operations. For instance, the Mars Perseverance rover, developed by Jet Propulsion Laboratory (JPL), extensively utilizes titanium and aluminum alloys in its chassis and arm structures. Looking forward, missions such as the European Space Agency’s (ESA) Rosalind Franklin rover and NASA’s VIPER lunar rover are expected to further optimize alloy compositions to balance mass, strength, and thermal performance.
Composite materials, including carbon fiber-reinforced polymers (CFRPs), are increasingly integrated into robotic hardware to achieve even greater weight reductions and enhanced resistance to temperature extremes and radiation. These composites are used in instrument housings, booms, and protective panels. Airbus, a key contractor for ESA’s ExoMars program, has pioneered the use of advanced composites in lander and rover components, enabling more robust and lightweight designs. The trend is set to continue, with upcoming lunar and Martian missions incorporating next-generation composites for both structural and shielding applications.
Additive manufacturing (AM), or 3D printing, is revolutionizing the fabrication of complex, mission-specific parts for robotic explorers. AM allows for rapid prototyping, reduced material waste, and the creation of intricate geometries that are difficult or impossible to achieve with traditional methods. NASA has successfully flight-qualified 3D-printed titanium and Inconel parts for use in landers and probes, and is actively expanding the use of AM for both metallic and polymeric components. Lockheed Martin and Northrop Grumman are also investing in additive manufacturing for structural and propulsion elements in upcoming lunar and deep-space missions.
In the next few years, the convergence of lightweight alloys, advanced composites, and additive manufacturing is expected to yield robotic space hardware that is lighter, stronger, and more adaptable than ever before. These innovations will be pivotal in supporting the increasing cadence and complexity of robotic missions to the Moon, Mars, and beyond.
Mission Pipeline: Upcoming Lunar, Martian, and Deep Space Deployments
The period from 2025 onward is poised to be a landmark era for robotic space exploration hardware, with a robust pipeline of missions targeting the Moon, Mars, and deep space. These missions are driven by a combination of national space agencies and private sector innovators, each deploying advanced rovers, landers, and probes to expand scientific knowledge and prepare for future human exploration.
On the lunar front, several missions are scheduled to deploy new generations of robotic hardware. NASA is advancing its Commercial Lunar Payload Services (CLPS) program, which will see multiple private companies deliver landers and scientific payloads to the Moon’s surface. Notably, Intuitive Machines and Astrobotic Technology are set to launch their Nova-C and Peregrine landers, respectively, carrying a suite of instruments and small rovers for surface exploration and resource prospecting. These missions are designed to test technologies for in-situ resource utilization and autonomous navigation, critical for sustained lunar presence.
Internationally, Japan Aerospace Exploration Agency (JAXA) is preparing for its Smart Lander for Investigating Moon (SLIM) mission, which aims to demonstrate pinpoint landing technology. Meanwhile, Indian Space Research Organisation (ISRO) is planning Chandrayaan-4, building on the success of Chandrayaan-3, with enhanced lander and rover capabilities for extended lunar surface operations.
Mars exploration will also see significant activity. European Space Agency (ESA) is targeting a 2028 launch for the Rosalind Franklin rover, part of the ExoMars program, which will drill beneath the Martian surface to search for signs of past life. While the timeline has shifted, hardware development continues, with a focus on advanced mobility and autonomous science operations. NASA’s Perseverance rover, already operational, will continue its sample caching activities, with plans for a Mars Sample Return campaign involving new landers and fetch rovers in the late 2020s.
In deep space, NASA’s Dragonfly mission, scheduled for launch in 2027, will deliver a rotorcraft lander to Saturn’s moon Titan, representing a leap in aerial robotic exploration. The mission’s hardware is designed to operate in Titan’s dense atmosphere, conducting surface and atmospheric analysis across multiple sites.
The outlook for robotic space exploration hardware in the coming years is characterized by increased international collaboration, rapid technological innovation, and a growing role for commercial providers. These missions will not only yield critical scientific data but also validate hardware and operational concepts essential for future human exploration beyond Earth.
Supply Chain & Integration: Challenges and Opportunities
The supply chain and integration landscape for robotic space exploration hardware—encompassing rovers, landers, and probes—faces both persistent challenges and emerging opportunities as missions intensify through 2025 and beyond. The complexity of these systems, combined with the stringent requirements for reliability, miniaturization, and radiation hardening, places unique demands on suppliers and integrators.
A primary challenge is the limited pool of qualified suppliers for critical components such as radiation-hardened electronics, high-performance actuators, and specialized materials. For instance, NASA and European Space Agency (ESA) missions often rely on a handful of established vendors for flight-qualified hardware, which can lead to bottlenecks and long lead times. The Mars Sample Return campaign, involving both NASA and ESA, has highlighted the need for robust supply chain coordination, as multiple international partners must synchronize deliveries of lander and rover subsystems.
Integration complexity is further heightened by the increasing modularity and autonomy of new robotic platforms. Companies like Airbus and Thales Group are developing modular lander and rover architectures to enable faster adaptation to mission-specific requirements. However, integrating subsystems from diverse suppliers—each with their own standards and testing protocols—can introduce compatibility risks and require extensive system-level validation.
Recent disruptions in global supply chains, including semiconductor shortages and geopolitical tensions, have underscored the vulnerability of space hardware programs to external shocks. In response, agencies and prime contractors are increasingly investing in dual-sourcing strategies and domestic manufacturing capabilities. For example, Lockheed Martin and Northrop Grumman are expanding in-house production of key avionics and propulsion components to mitigate reliance on single-source suppliers.
On the opportunity side, the rise of commercial lunar and planetary missions is fostering a more diverse supplier ecosystem. Companies such as Astrobotic Technology and Intuitive Machines are leveraging commercial-off-the-shelf (COTS) components and agile integration practices to reduce costs and accelerate timelines. This trend is expected to continue as NASA’s Commercial Lunar Payload Services (CLPS) program and similar initiatives in Europe and Asia open new markets for subsystem providers.
Looking ahead, digital engineering and model-based systems engineering (MBSE) are poised to streamline integration and verification processes. By enabling virtual prototyping and early detection of interface issues, these approaches can help mitigate risks associated with multi-vendor supply chains. As the cadence of robotic missions increases through 2025 and beyond, the ability to rapidly integrate, test, and qualify hardware from a global network of suppliers will be a key differentiator for both established aerospace primes and emerging commercial players.
Regulatory & Standards Landscape: International Collaboration and Compliance (e.g., ieee.org, asme.org)
The regulatory and standards landscape for robotic space exploration hardware—encompassing rovers, landers, and probes—continues to evolve rapidly as international missions proliferate and private sector participation increases. In 2025 and the coming years, the focus is on harmonizing technical standards, ensuring interoperability, and fostering responsible conduct in space through international collaboration.
Key international bodies such as the IEEE and the ASME play pivotal roles in developing and updating standards relevant to space robotics. The IEEE, for example, maintains standards for robotic systems’ safety, communication protocols, and software reliability, which are increasingly referenced in mission requirements for both governmental and commercial projects. ASME contributes with standards for mechanical systems, materials, and testing procedures, ensuring that hardware can withstand the harsh conditions of space environments.
The United Nations Committee on the Peaceful Uses of Outer Space (COPUOS) continues to provide a framework for international cooperation, emphasizing the need for transparency, data sharing, and the peaceful use of outer space. In 2025, COPUOS is expected to further discussions on guidelines for the mitigation of space debris and the protection of planetary environments, both of which directly impact the design and operation of robotic explorers.
Space agencies such as NASA, the European Space Agency (ESA), and JAXA are increasingly aligning their mission requirements with international standards to facilitate joint missions and technology sharing. For instance, NASA’s Artemis program and ESA’s ExoMars rover have both adopted standardized interfaces and communication protocols to enable cross-agency hardware integration and data exchange. This trend is expected to accelerate as more countries and private entities, such as ispace, inc. and Astrobotic Technology, Inc., prepare to deploy landers and rovers to the Moon and Mars.
Looking ahead, the next few years will likely see the formalization of new standards addressing autonomy, AI-driven decision-making, and cybersecurity for robotic space hardware. The IEEE and ASME are anticipated to release updated guidelines reflecting advances in these areas, while international forums will continue to address the ethical and legal implications of autonomous exploration. As the sector grows, compliance with these evolving standards will be essential for mission success, risk mitigation, and the advancement of collaborative exploration beyond Earth.
Investment & Funding Trends: Government, Commercial, and Venture Capital
Investment and funding in robotic space exploration hardware—encompassing rovers, landers, and probes—are experiencing a dynamic phase as both government and commercial actors intensify their activities. In 2025, government agencies remain the primary financiers of large-scale missions, but commercial and venture capital (VC) participation is growing, particularly in lunar and planetary exploration.
Government funding continues to underpin flagship missions. The National Aeronautics and Space Administration (NASA) maintains robust investment in Mars and lunar exploration, with ongoing support for the Mars Sample Return campaign and the Artemis program’s robotic precursors. NASA’s Commercial Lunar Payload Services (CLPS) initiative, which contracts private companies to deliver payloads to the Moon, has awarded hundreds of millions of dollars to firms such as Intuitive Machines, Astrobotic Technology, and Firefly Aerospace. These contracts are designed to stimulate the commercial lunar lander market and foster a sustainable lunar economy.
Internationally, the European Space Agency (ESA) and Japan Aerospace Exploration Agency (JAXA) are investing in next-generation robotic missions, such as ESA’s Rosalind Franklin Mars rover and JAXA’s lunar and asteroid probes. China’s National Space Administration (CNSA) is also allocating significant state resources to its Chang’e lunar program and Tianwen planetary missions, with plans for further robotic landers and sample return efforts.
On the commercial side, investment is increasingly driven by lunar surface access and in-situ resource utilization (ISRU) opportunities. Companies like ispace (Japan) and Draper (US) have secured both government contracts and private funding rounds to develop landers and small rovers. The growing interest in lunar infrastructure is attracting venture capital, with several startups raising Series A and B rounds to support hardware development and mission integration.
Venture capital activity, while still modest compared to satellite and launch sectors, is on the rise. Investors are targeting companies with dual-use technologies—such as autonomous navigation, miniaturized sensors, and modular lander platforms—that can serve both government and commercial customers. The maturation of lunar delivery services and the prospect of Mars and asteroid missions are expected to further catalyze private investment through 2025 and beyond.
Looking ahead, the interplay between government anchor contracts and private capital is likely to accelerate innovation and reduce costs in robotic exploration hardware. As more missions transition from demonstration to operational phases, the sector is poised for increased deal flow and strategic partnerships, particularly in support of lunar and planetary surface operations.
Future Outlook: Emerging Markets, Next-Gen Concepts, and Long-Term Impact
The period from 2025 onward is poised to be transformative for robotic space exploration hardware, with a surge in missions, new market entrants, and technological advancements. The global focus is shifting toward more ambitious planetary science, lunar resource prospecting, and interplanetary reconnaissance, driving demand for next-generation rovers, landers, and probes.
In 2025, several high-profile missions are scheduled to deploy advanced robotic hardware. NASA is preparing for the launch of the VIPER lunar rover, designed to prospect for water ice at the Moon’s south pole, a key step for future lunar habitation and resource utilization. The agency is also advancing the Mars Sample Return campaign, which will rely on a new lander and fetch rover to collect and return Martian soil to Earth. Meanwhile, European Space Agency (ESA) is progressing with the Rosalind Franklin ExoMars rover, targeting a 2028 launch after technical delays, with a focus on subsurface life detection on Mars.
Commercial players are increasingly shaping the landscape. ispace, a Japanese lunar exploration company, is developing a series of lunar landers and rovers under its HAKUTO-R program, with missions planned through the late 2020s. Astrobotic Technology and Intuitive Machines, both U.S.-based, are contracted under NASA’s Commercial Lunar Payload Services (CLPS) initiative to deliver landers and small rovers to the Moon, supporting both scientific and commercial payloads. These missions are expected to catalyze a new market for lunar surface delivery and in-situ resource utilization hardware.
Technological trends include increased autonomy, modularity, and miniaturization. Next-gen rovers and landers are being designed with advanced AI for navigation and decision-making, enabling more complex operations with less reliance on Earth-based control. Modular hardware platforms are emerging, allowing for rapid reconfiguration and adaptation to diverse mission profiles. Additionally, the use of lightweight composite materials and additive manufacturing is reducing mass and cost, making frequent and distributed exploration feasible.
Looking ahead, the convergence of public and private investment, coupled with international collaboration, is expected to accelerate the deployment of robotic explorers across the Moon, Mars, and beyond. The proliferation of commercial lunar landers and the maturation of autonomous rover technologies will likely open new markets in resource prospecting, infrastructure deployment, and scientific research, fundamentally reshaping the economics and scope of planetary exploration.
Sources & References
- NASA
- European Space Agency (ESA)
- Astrobotic Technology
- ispace
- Japan Aerospace Exploration Agency (JAXA)
- DLR
- Indian Space Research Organisation (ISRO)
- Maxar Technologies
- NASA
- European Space Agency (ESA)
- Airbus
- Lockheed Martin
- Northrop Grumman
- Thales Group
- IEEE
- ASME
- National Space Administration