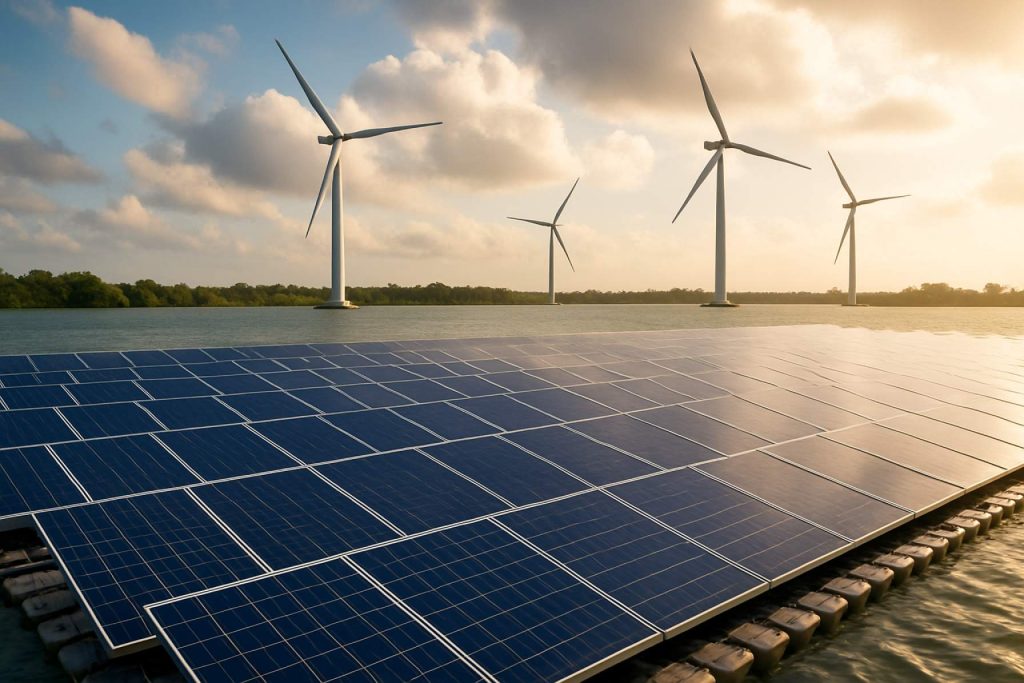
- High-temperature fuel cells, such as solid oxide and molten carbonate types, operate above 600°C and efficiently convert hydrogen or natural gas into electricity with minimal emissions.
- The global high-temperature fuel cell market is projected to grow rapidly, with a nearly 26% compound annual growth rate through 2031, fueled by the push for decarbonization and energy security.
- Advantages include direct electricity generation, high efficiency, integration with combined heat and power systems, and suitability for distributed grids and heavy transport.
- Major challenges remain, including high production costs, underdeveloped supply chains, complex thermal management, and uneven regional regulation and hydrogen infrastructure.
- Key industry leaders (Siemens Energy, Bosch, GE, Mitsubishi Heavy Industries) and innovative projects in the U.S., Germany, and Asia-Pacific are accelerating technology development and adoption.
- Mastering high-temperature fuel cell science and logistics is critical for achieving a sustainable, decarbonized global energy sector.
Molten metal glimmers at the heart of industrial ambition. In laboratories from California to Bavaria, a wave of scientific ingenuity pulses through the high-temperature fuel cell market, forging a bold path toward a world less reliant on carbon. These advanced devices, operating at temperatures soaring beyond 600°C, harness hydrogen or natural gas to produce not just electricity but also hope for a cleaner future.
Wires hum and turbines spin as solid oxide fuel cells and their molten carbonate cousins take center stage. Once niche technologies reserved for space programs and universities, high-temperature fuel cells now surge at a breathtaking pace—set for a compound annual growth rate just shy of 26% until 2031. This acceleration rides a wave of global urgency: energy producers and policymakers hunt for ways to slash greenhouse gas emissions and harden the world’s resilience against unstable fossil fuel supplies.
What sets high-temperature fuel cells apart? Pure efficiency. Their ability to convert the chemical energy of fuel directly into electricity—skipping the noisy, wasteful steps of combustion—makes them the darling of both heavy industry and avant-garde power grids. They slip seamlessly into distributed generation, fuel buses and trucks of tomorrow, and, most strikingly, can pair with combined heat and power systems to wring every watt from each molecule of hydrogen.
Yet, for all their promise, the path forward is not simple. The roar of progress must compete with the cold math of economics—production costs remain steep, and the supply chains for crucial materials have yet to mature into a robust backbone. Thermal management, always a challenge in the world of extreme temperatures, demands relentless innovation.
Investors contend with a landscape where regulations zig and zag between regions. The hydrogen infrastructure—pipelines, storage, filling stations—remains patchy at best, even as companies sprint to build it out. Governments, sensing opportunity and necessity, deploy incentives and subsidies, fueling research and reducing barriers. The United States and Germany—both laboratories and battlegrounds for energy innovation—lead with audacious projects, such as the 280-megawatt green hydrogen initiative in Emden that aims to erase up to 800,000 tons of CO2 from steelmaking each year.
Meanwhile, alliances form at the speed of technical progress. Bloom Energy’s collaboration with AI powerhouse CoreWeave, Inc. signals the expanding appetite for reliable, scalable clean power in a digital age. Siemens Energy, Bosch, GE, and Mitsubishi Heavy Industries jostle for position, pushing the envelope on durability, scale, and integration.
Europe and North America spearhead adoption, leveraging mature industrial bases and strong policy frameworks. Asia-Pacific is catching up, with China, Japan, and South Korea investing billions in infrastructure and R&D. In every region, the promise is tantalizing: energy security, environmental stewardship, and a new industry blossoming around the cleanest molecules known.
As the molten cores of tomorrow’s power plants pulse hotter and greener, the central message crystallizes—decarbonizing the global economy will hinge on mastering both the science and logistics of high-temperature fuel cells. Companies and countries willing to invest in innovation, to push past cost and complexity, may find themselves not just powering their own homes and factories, but also lighting the way for a sustainable planet.
Explore more about the future of clean energy at Bloom Energy and Siemens Energy.
Molten Power Revolution: 12 Insider Secrets About High-Temperature Fuel Cells the Industry Isn’t Telling You
High-Temperature Fuel Cells: The Full Story Unveiled
High-temperature fuel cells (HTFCs), including solid oxide fuel cells (SOFCs) and molten carbonate fuel cells (MCFCs), are gathering momentum in the global shift toward low-carbon energy. While the source article provides an inspiring overview, there’s much more beneath the molten surface. Here’s an authoritative, research-backed exploration for innovators, businesses, and future-focused individuals.
What You Need to Know: Essential Facts & FAQs
1. Latest Features, Specs, and Pricing Insights
– Efficiency: SOFCs can achieve electrical efficiencies up to 60% and, when paired in combined heat and power (CHP) units, overall system efficiencies can exceed 85%. (Source: U.S. Department of Energy)
– Fuel Flexibility: Both SOFCs and MCFCs can operate on hydrogen, natural gas, biogas, and even ammonia, making them adaptable to changing fuel markets.
– Output Range: Systems span from small 1-kW residential models to multi-megawatt industrial plants.
– Pricing: While costs are declining, current commercial SOFC systems can range from $4,500 to $7,000 per kilowatt (kW) installed, though this is expected to fall as economies of scale improve. For comparison, conventional natural gas turbines average $1,000–$1,500/kW. (Source: International Energy Agency)
2. Security, Durability & Sustainability
– Materials: Key components include ceramics and exotic alloys that can withstand 600–1000°C. Innovations in nickel or cobalt-free electrodes are improving security against resource constraints (Nature, 2023).
– Lifespan: Best-in-class SOFC stacks now last 40,000–80,000 hours (4.5–9 years of continuous use).
– Recycling: End-of-life recycling programs are emerging for ceramics and precious metals—an important step toward a truly circular clean energy economy.
3. Real-World Use Cases & Industry Trends
– Data Centers: Bloom Energy’s installations at tech firms provide uninterrupted clean power for mission-critical applications, with minimal emissions.
– Heavy Industry: Steel and cement producers use fuel cells to cut direct CO2 emissions—for example, Germany’s Thyssenkrupp pilots using SOFCs with green hydrogen.
– Electric Mobility: Pilot hydrogen fuel cell buses and trucks, notably in Japan and California, leverage SOFC range and quick refueling.
– Microgrids: Hospitals and universities deploy HTFCs for resilient, off-grid power with combined heat and power (CHP) benefits.
4. Market Forecasts & Predicted Growth
– Market Size: Valued at roughly $1.8 billion in 2023, with forecasts exceeding $10 billion by 2031, reflecting a 26% compound annual growth rate. (Source: MarketsandMarkets)
– Regional Leaders: Europe (esp. Germany, UK), North America (U.S., Canada), and East Asia (Japan, S. Korea, China) dominate R&D and deployment.
5. How-To Steps & Implementation Hacks
– Beginning an HTFC Project:
1. Conduct a feasibility study: Assess energy needs, fuel availability, and regulatory requirements.
2. Select a system type: SOFCs for higher efficiency and fuel flexibility; MCFCs for high capacity.
3. Navigate Permitting: Work with local authorities to streamline installation approvals.
4. Integrate with CHP: For maximum energy extraction, design your site to capture and use waste heat.
5. Plan for maintenance: Schedule regular stack monitoring and replacement cycles.
– Life Hack: Approach local clean energy incentive programs; many regions offer grants covering up to 50% of upfront costs (check policies in U.S. and EU).
6. Reviews, Comparisons & Expert Insights
– SOFC vs. MCFC:
– SOFC: Higher efficiency, broader fuel options, but more sensitive to thermal cycling.
– MCFC: Slightly lower efficiency, excels in large-scale industrial settings, tolerant to CO₂ in fuel streams.
– Leading Brands: Bloom Energy and Siemens Energy are global leaders, known for reliability, capacity, and innovation.
– User Reviews: Early adopters report substantial OPEX savings at scale, but note challenges in stack replacement intervals and technical support.
7. Controversies, Limitations & Challenges
– High upfront cost: Commercial maturity is still evolving, though costs are dropping with mass production.
– Material Bottlenecks: SOFCs’ reliance on rare metals like yttria and scandia is pushing R&D toward abundant alternatives.
– Hydrogen Infrastructure: The “hydrogen gap” is real—clean, affordable hydrogen supply and storage remain limiting factors in many markets.
– Start-Up Time: SOFCs take hours to reach operating temperature, making them less suitable for rapid-response peaking power.
8. Security, Compatibility & Integration
– Cybersecurity: Digital integration with grid and industrial systems requires robust endpoint protection—especially as fuel cell systems become targets for hackers.
– Compatibility: HTFCs can complement renewables, acting as baseload (always-on) generators when solar and wind are intermittent.
9. Pros & Cons At a Glance
Pros:
– High efficiency and low emissions (especially when using green hydrogen)
– Fuel flexibility and CHP suitability
– Stable, silent, vibration-free operation
Cons:
– High capital cost and material supply risks
– Complex thermal management and slow start-up/shutdown
– Limited hydrogen infrastructure
10. Industry-Leading Expert Opinions
– The Hydrogen Council and IEA both cite HTFCs as “essential for deep decarbonization” in sectors like chemicals, heavy transport, and district heating.
– McKinsey notes that as carbon pricing tightens and green hydrogen scales, economic parity versus fossil generation is plausible by 2030.
Actionable Recommendations & Quick Tips
– For Businesses: Start piloting HTFC-powered microgrids in regions with high grid prices or prone to outages; seize government incentives early.
– For Investors: Prioritize companies innovating on stack lifetimes and alternative materials—this will decide long-term profitability.
– For Policymakers: Support hydrogen infrastructure build-out and foster clear, stable regulatory frameworks to attract more investment.
– For Homeowners: Keep an eye on coming 1-5kW SOFC home units as costs drop—early adoption in high-electricity-cost regions is becoming viable.
Curious for more? Dive into proven solutions at Bloom Energy or explore industrial-scale energy integration at Siemens Energy.
The molten core of clean energy innovation is heating up—the time to act is now.