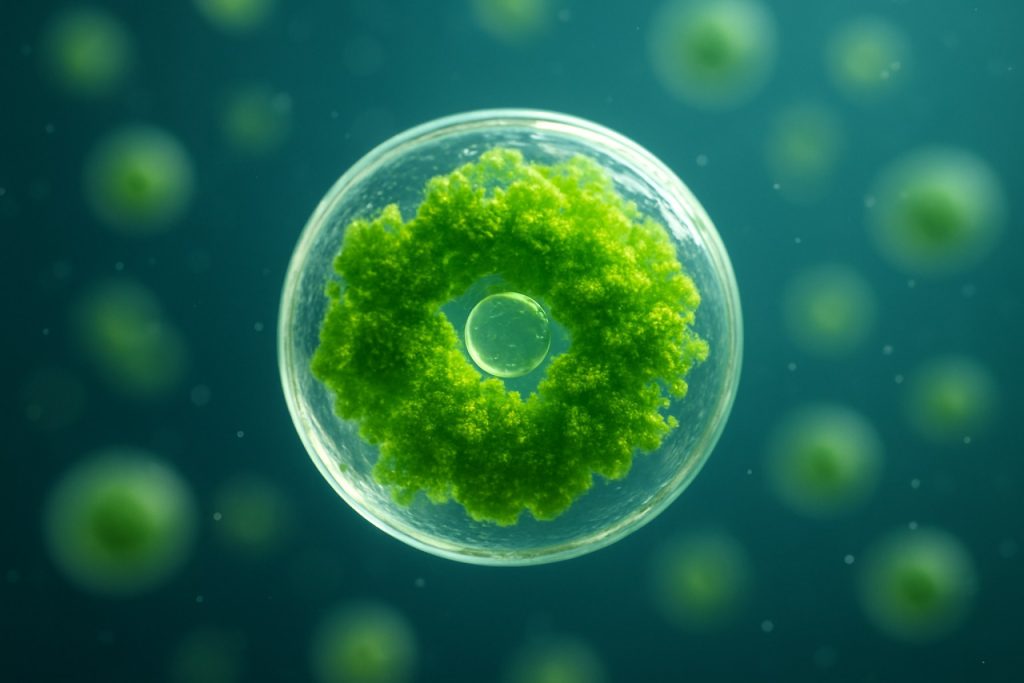
The Science Behind DHA Biosynthesis in Marine Microalgae: Unveiling Nature’s Omega-3 Factory and Its Biotechnological Promise
- Introduction to DHA and Its Biological Importance
- Overview of Marine Microalgae as DHA Producers
- Key Enzymes in the DHA Biosynthetic Pathway
- Genetic Regulation of DHA Synthesis in Microalgae
- Environmental Factors Influencing DHA Production
- Comparative Analysis: Microalgal vs. Fish-Derived DHA
- Metabolic Engineering for Enhanced DHA Yields
- Industrial Cultivation and Harvesting Techniques
- Applications in Nutrition, Pharmaceuticals, and Aquaculture
- Future Directions and Emerging Research in DHA Biosynthesis
- Sources & References
Introduction to DHA and Its Biological Importance
Docosahexaenoic acid (DHA; 22:6 n-3) is a long-chain polyunsaturated fatty acid (PUFA) that plays a critical role in the structure and function of cellular membranes, particularly in the brain, retina, and other neural tissues. As an omega-3 fatty acid, DHA is essential for human health, contributing to cognitive development, visual acuity, and cardiovascular function. While DHA can be obtained through dietary sources such as fish and seafood, its primary origin in marine ecosystems is the biosynthetic activity of microalgae. These unicellular photosynthetic organisms are the foundational producers of DHA, which then accumulates up the food chain through zooplankton and fish consumption.
The biological importance of DHA is underscored by its high concentration in the phospholipid bilayers of neuronal and retinal cells, where it modulates membrane fluidity, signal transduction, and synaptic function. In humans, adequate DHA intake is associated with improved neurodevelopment in infants, maintenance of cognitive function in adults, and reduced risk of chronic diseases such as coronary heart disease. The World Health Organization and other health authorities recognize DHA as a vital nutrient, recommending its inclusion in infant formulas and dietary supplements, especially for populations with limited access to marine foods.
Marine microalgae synthesize DHA via complex metabolic pathways involving a series of desaturation and elongation reactions. Unlike higher plants and most terrestrial organisms, which lack the enzymatic machinery to produce significant amounts of long-chain omega-3 PUFAs, certain microalgal species—such as those belonging to the genera Schizochytrium, Crypthecodinium, and Isochrysis—are highly efficient at DHA biosynthesis. These microalgae utilize either the conventional aerobic pathway, which involves Δ6-desaturase and elongase enzymes, or alternative anaerobic polyketide synthase (PKS) pathways, depending on the species. The diversity of biosynthetic routes among microalgae reflects evolutionary adaptations to varying ecological niches and environmental conditions in marine habitats.
The significance of microalgal DHA biosynthesis extends beyond marine ecology; it has profound implications for sustainable nutrition and biotechnology. As global demand for omega-3 fatty acids increases, microalgae offer a renewable and environmentally friendly source of DHA, circumventing the limitations of wild fisheries and supporting the development of vegetarian and vegan supplements. Organizations such as the Food and Agriculture Organization of the United Nations have highlighted the potential of microalgae in addressing nutritional needs and promoting food security through innovative aquaculture and bioprocessing technologies.
Overview of Marine Microalgae as DHA Producers
Marine microalgae are recognized as primary producers of docosahexaenoic acid (DHA), a long-chain omega-3 polyunsaturated fatty acid essential for human health, particularly for neural and visual development. Unlike higher plants, which generally lack the enzymatic machinery to synthesize DHA, certain marine microalgae possess specialized biosynthetic pathways that enable the efficient production of this vital nutrient. These microalgae serve as the foundational source of DHA in marine food webs, with higher trophic organisms, such as fish, accumulating DHA through their diet of microalgae and microalgae-consuming zooplankton.
The diversity of marine microalgae is vast, encompassing groups such as diatoms, dinoflagellates, and thraustochytrids, many of which are prolific DHA producers. Notably, thraustochytrids (heterotrophic protists often grouped with microalgae for their DHA productivity) and certain species of the genera Schizochytrium and Crypthecodinium are commercially exploited for DHA production. These organisms are cultivated in controlled environments to produce DHA-rich biomass, which is then processed into nutritional supplements and food additives. The ability of microalgae to synthesize DHA de novo, using carbon dioxide, light (in phototrophic species), and simple nutrients, makes them a sustainable and scalable alternative to traditional fish oil sources.
The biosynthesis of DHA in marine microalgae involves complex enzymatic pathways, primarily the polyketide synthase (PKS) pathway and the conventional fatty acid elongation and desaturation pathway. The PKS pathway, found in thraustochytrids, is particularly efficient and distinct from the pathways present in higher plants and animals. This metabolic versatility allows microalgae to accumulate high levels of DHA under optimized cultivation conditions, such as specific temperature, salinity, and nutrient regimes. Advances in algal biotechnology have further enhanced DHA yields through strain selection, metabolic engineering, and process optimization.
Given the growing demand for sustainable omega-3 sources, marine microalgae have attracted significant attention from both scientific and industrial communities. Organizations such as the Food and Agriculture Organization of the United Nations and the World Health Organization recognize the importance of microalgae-derived DHA in addressing nutritional needs and reducing pressure on marine fisheries. Furthermore, companies specializing in algal biotechnology, such as those affiliated with the Algae Biomass Organization, are at the forefront of commercial DHA production, promoting microalgae as a reliable and environmentally friendly source of this essential fatty acid.
Key Enzymes in the DHA Biosynthetic Pathway
Docosahexaenoic acid (DHA, 22:6n-3) is a long-chain polyunsaturated fatty acid (LC-PUFA) essential for human health, and marine microalgae are among the primary natural producers of DHA in aquatic ecosystems. The biosynthesis of DHA in marine microalgae involves a complex series of enzymatic reactions, primarily through the aerobic desaturase/elongase pathway, also known as the “Sprecher pathway.” This pathway is characterized by the sequential action of key enzymes that introduce double bonds and elongate the carbon chain of precursor fatty acids.
The process typically begins with α-linolenic acid (ALA, 18:3n-3) as the substrate. The first critical enzyme is Δ6-desaturase, which introduces a double bond at the Δ6 position, converting ALA to stearidonic acid (SDA, 18:4n-3). This is followed by elongation via an elongase enzyme, producing eicosatetraenoic acid (ETA, 20:4n-3). The next step involves Δ5-desaturase, which converts ETA to eicosapentaenoic acid (EPA, 20:5n-3), another important omega-3 fatty acid.
To synthesize DHA, EPA undergoes further elongation by a C2-elongase to form docosapentaenoic acid (DPA, 22:5n-3). Subsequently, Δ4-desaturase introduces a double bond at the Δ4 position, yielding DHA. In some microalgal species, an alternative “Δ6 pathway” or a polyketide synthase (PKS) pathway may also contribute to DHA biosynthesis, particularly in thraustochytrids and certain dinoflagellates. The PKS pathway is distinct in that it does not rely on the conventional desaturase/elongase system but instead utilizes a modular enzyme complex similar to those found in antibiotic biosynthesis.
The genes encoding these key enzymes—Δ6-desaturase, Δ5-desaturase, Δ4-desaturase, and elongases—are tightly regulated and often present in multiple isoforms, reflecting the diversity of microalgal species and their adaptation to varying environmental conditions. The activity and expression of these enzymes are influenced by factors such as temperature, light, and nutrient availability, which can modulate the overall DHA yield in microalgal cultures.
Research into the molecular biology and biochemistry of these enzymes has been instrumental in advancing metabolic engineering efforts aimed at enhancing DHA production in both native and heterologous hosts. Organizations such as the National Institute of Genetics and the Japan Agency for Marine-Earth Science and Technology have contributed to the elucidation of these pathways, supporting the development of sustainable sources of DHA for nutritional and pharmaceutical applications.
Genetic Regulation of DHA Synthesis in Microalgae
The genetic regulation of docosahexaenoic acid (DHA) synthesis in marine microalgae is a complex process involving multiple enzymatic pathways and regulatory networks. DHA, a long-chain omega-3 polyunsaturated fatty acid, is primarily synthesized in marine microalgae through the polyketide synthase (PKS) pathway or the conventional fatty acid elongation and desaturation pathway. The choice of pathway and its regulation are species-dependent, with thraustochytrids and certain dinoflagellates favoring the PKS route, while other microalgae such as some diatoms utilize the elongase/desaturase pathway.
Key enzymes involved in the elongase/desaturase pathway include Δ6-desaturase, Δ5-desaturase, and elongases, which sequentially convert shorter-chain fatty acids into DHA. The expression of genes encoding these enzymes is tightly regulated at the transcriptional and post-transcriptional levels. Environmental factors such as light intensity, temperature, and nutrient availability can modulate gene expression, thereby influencing DHA yield. For example, nitrogen limitation has been shown to upregulate genes involved in lipid biosynthesis, including those responsible for DHA production.
Recent advances in genomics and transcriptomics have enabled the identification of gene clusters and regulatory elements associated with DHA biosynthesis. Promoter analysis has revealed the presence of specific cis-acting elements that respond to environmental cues, allowing microalgae to fine-tune DHA synthesis in response to changing conditions. Additionally, transcription factors such as basic helix-loop-helix (bHLH) and MYB families have been implicated in the regulation of fatty acid biosynthetic genes.
Genetic engineering approaches have further elucidated the regulatory mechanisms underlying DHA synthesis. Overexpression of key desaturase and elongase genes has resulted in enhanced DHA accumulation in model microalgal species. Conversely, gene knockout studies have confirmed the essential roles of these enzymes in the biosynthetic pathway. The application of CRISPR/Cas9 genome editing has accelerated functional genomics studies, enabling precise manipulation of regulatory genes to optimize DHA production.
International organizations such as the Food and Agriculture Organization of the United Nations and research consortia like the European Marine Biological Resource Centre support research into marine microalgal genetics and biotechnology. These efforts aim to harness the genetic potential of microalgae for sustainable DHA production, contributing to global nutrition and aquaculture industries.
Environmental Factors Influencing DHA Production
The biosynthesis of docosahexaenoic acid (DHA) in marine microalgae is a complex process that is highly sensitive to a range of environmental factors. These factors not only influence the growth and metabolic activity of microalgae but also directly affect the yield and composition of DHA, a long-chain omega-3 polyunsaturated fatty acid of significant nutritional and pharmaceutical value.
One of the primary environmental determinants is light intensity and quality. Microalgae utilize light as an energy source for photosynthesis, and both the amount and wavelength of light can modulate lipid metabolism. Studies have shown that suboptimal or excessive light can reduce DHA accumulation, while specific light regimes may enhance its biosynthesis by altering the expression of key enzymes in the fatty acid pathway. The National Aeronautics and Space Administration (NASA) has supported research into optimizing light conditions for algal growth in controlled environments, highlighting the importance of light management in maximizing DHA yields.
Temperature is another critical factor. Generally, lower cultivation temperatures favor the accumulation of polyunsaturated fatty acids like DHA, as microalgae adjust their membrane fluidity in response to cold by increasing unsaturated lipid content. However, extremely low temperatures can inhibit overall growth, necessitating a balance between optimal biomass production and DHA enrichment. The Food and Agriculture Organization of the United Nations (FAO) recognizes temperature as a key parameter in algal biotechnology, especially for large-scale cultivation systems.
Nutrient availability, particularly nitrogen and phosphorus, also plays a pivotal role. Limitation of these nutrients often triggers lipid accumulation as a stress response, but the effect on DHA content can vary depending on the microalgal species and cultivation strategy. Some species increase total lipid content under nutrient stress, but the proportion of DHA may decrease if the stress is too severe or prolonged. The United Nations Environment Programme (UNEP) has emphasized the need for sustainable nutrient management in aquaculture and algal production to balance productivity with environmental impact.
Other factors such as salinity, pH, and dissolved oxygen further influence DHA biosynthesis. For instance, certain marine microalgae require specific salinity ranges to maintain cellular homeostasis and optimal enzyme activity for fatty acid synthesis. Fluctuations in pH and oxygen levels can also affect metabolic fluxes and the efficiency of DHA production.
In summary, the interplay of light, temperature, nutrients, and other abiotic factors governs the efficiency of DHA biosynthesis in marine microalgae. Understanding and optimizing these environmental parameters is essential for enhancing DHA yields in both research and commercial production settings, as recognized by leading international organizations and scientific bodies.
Comparative Analysis: Microalgal vs. Fish-Derived DHA
Docosahexaenoic acid (DHA) is a long-chain omega-3 polyunsaturated fatty acid essential for human health, particularly for neural development and cardiovascular function. Traditionally, fish and fish oils have been the primary dietary sources of DHA. However, the original biosynthetic source of DHA in marine ecosystems is not fish, but marine microalgae. Fish accumulate DHA through their diet, primarily by consuming microalgae or organisms that have fed on microalgae. This section provides a comparative analysis of DHA derived from marine microalgae versus that obtained from fish, focusing on biosynthetic pathways, sustainability, purity, and nutritional equivalence.
Marine microalgae synthesize DHA de novo via two main biosynthetic pathways: the aerobic desaturase/elongase pathway and the anaerobic polyketide synthase (PKS) pathway. The former involves a series of desaturation and elongation steps converting shorter-chain fatty acids into DHA, while the latter is a more direct route found in certain thraustochytrids and other protists. In contrast, fish do not possess the enzymatic machinery to synthesize significant amounts of DHA; instead, they bioaccumulate it from their diet. Thus, microalgae represent the foundational producers of DHA in marine food webs (Food and Agriculture Organization of the United Nations).
From a sustainability perspective, microalgal DHA production offers several advantages over fish-derived sources. Overfishing and environmental concerns have raised questions about the long-term viability of fish oil as a DHA source. In contrast, microalgae can be cultivated in controlled environments, independent of marine ecosystems, reducing pressure on wild fish stocks and minimizing ecological impact. Additionally, microalgal cultivation can utilize non-arable land and brackish or seawater, further enhancing its sustainability profile (United Nations Environment Programme).
Purity and safety are also critical considerations. Fish oils may contain environmental contaminants such as heavy metals, dioxins, and polychlorinated biphenyls (PCBs) due to bioaccumulation in marine food chains. Microalgal DHA, produced in closed bioreactors, is less susceptible to such contamination, resulting in a purer end product. This makes microalgal DHA particularly attractive for infant formulas, nutraceuticals, and vegan or vegetarian consumers (European Food Safety Authority).
Nutritionally, DHA from microalgae is chemically identical to that from fish, ensuring equivalent bioavailability and physiological benefits. Regulatory authorities, including the U.S. Food and Drug Administration and European Food Safety Authority, recognize microalgal DHA as a safe and effective alternative to fish-derived DHA for human consumption.
Metabolic Engineering for Enhanced DHA Yields
Metabolic engineering has emerged as a pivotal strategy to enhance docosahexaenoic acid (DHA) yields in marine microalgae, which are among the primary natural producers of this essential omega-3 fatty acid. DHA biosynthesis in microalgae typically proceeds via the polyketide synthase (PKS) pathway or the conventional fatty acid elongation and desaturation pathway, depending on the species. However, natural DHA yields are often insufficient for commercial applications, prompting extensive research into genetic and metabolic modifications to boost productivity.
Key targets for metabolic engineering include the upregulation of genes encoding desaturases and elongases, which are critical enzymes in the conversion of shorter-chain fatty acids to DHA. For example, overexpression of Δ6-desaturase and Δ5-elongase has been shown to increase the flux toward DHA in several microalgal species. Additionally, the introduction of heterologous genes from other DHA-producing organisms can further enhance the biosynthetic capacity. Advanced genome editing tools such as CRISPR/Cas9 and TALENs have facilitated precise modifications, enabling the knockout of competing pathways and the optimization of regulatory elements to favor DHA accumulation.
Another important aspect of metabolic engineering is the optimization of central carbon metabolism to ensure sufficient precursor supply for fatty acid synthesis. Redirecting carbon flux from carbohydrate or protein synthesis toward lipid biosynthesis can significantly improve DHA yields. This is often achieved by manipulating key metabolic nodes, such as acetyl-CoA carboxylase and malic enzyme, which play central roles in providing substrates for fatty acid elongation.
Efforts are also underway to enhance the tolerance of microalgae to high intracellular lipid concentrations, which can otherwise inhibit growth and productivity. Engineering robust strains capable of thriving under industrial cultivation conditions is essential for scalable DHA production. Furthermore, the use of systems biology approaches, including transcriptomics, proteomics, and metabolomics, allows for the comprehensive mapping of metabolic networks and the identification of novel engineering targets.
Several organizations and research consortia are actively involved in advancing metabolic engineering for DHA production in microalgae. For instance, the National Renewable Energy Laboratory (NREL) in the United States conducts research on algal bioengineering, while the European Food Safety Authority (EFSA) provides regulatory guidance on the safety of genetically modified microalgae-derived products. These efforts collectively aim to make sustainable, high-yield DHA production a commercial reality, supporting both nutritional and industrial applications.
Industrial Cultivation and Harvesting Techniques
The industrial cultivation and harvesting of marine microalgae for docosahexaenoic acid (DHA) production is a rapidly advancing field, driven by the growing demand for sustainable sources of omega-3 fatty acids. Marine microalgae, such as species from the genera Schizochytrium, Crypthecodinium, and Isochrysis, are recognized as primary producers of DHA in aquatic ecosystems. Their ability to synthesize high levels of DHA makes them attractive for commercial exploitation, particularly as an alternative to fish oil.
Industrial-scale cultivation of DHA-rich microalgae typically employs two main systems: open ponds and closed photobioreactors. Open pond systems, such as raceway ponds, are cost-effective and suitable for large-scale production, but are susceptible to contamination and environmental fluctuations. In contrast, closed photobioreactors offer greater control over growth conditions, including light, temperature, and nutrient supply, resulting in higher product consistency and reduced risk of contamination. However, these systems are more capital-intensive and require advanced engineering solutions to optimize light penetration and gas exchange.
The selection of cultivation parameters is critical for maximizing DHA yield. Factors such as light intensity, temperature, salinity, and nutrient composition are carefully optimized to promote algal growth and lipid accumulation. For example, nitrogen limitation is a common strategy to trigger lipid biosynthesis, including DHA, in many microalgal species. Additionally, the use of heterotrophic cultivation—where microalgae are grown in the dark using organic carbon sources—has been shown to significantly enhance DHA productivity in certain strains, such as Schizochytrium spp.
Harvesting microalgal biomass presents unique challenges due to the small size and dilute concentration of cells in culture. Common harvesting techniques include centrifugation, flocculation, filtration, and sedimentation. The choice of method depends on the specific microalgal species, cell density, and downstream processing requirements. After harvesting, the biomass is typically subjected to drying and cell disruption processes to facilitate the extraction of DHA-rich oils.
The industrial production of DHA from microalgae is subject to regulatory oversight to ensure product safety and quality. Organizations such as the U.S. Food and Drug Administration and the European Food Safety Authority provide guidelines for the approval and monitoring of microalgal-derived DHA products, particularly for use in infant formula and dietary supplements. Furthermore, research institutions and industry consortia, including the Algae Industry Magazine, play a key role in disseminating best practices and technological advancements in the field.
Applications in Nutrition, Pharmaceuticals, and Aquaculture
Docosahexaenoic acid (DHA) is a long-chain omega-3 polyunsaturated fatty acid that plays a critical role in human health, particularly in brain development, cardiovascular function, and visual acuity. Marine microalgae are the primary natural producers of DHA in aquatic ecosystems, serving as the foundational source of this essential fatty acid for higher trophic levels, including fish and, ultimately, humans. The biosynthesis of DHA in marine microalgae has enabled a range of applications across nutrition, pharmaceuticals, and aquaculture, offering sustainable alternatives to traditional fish oil sources.
In human nutrition, DHA is recognized for its importance in prenatal and infant development, as well as in maintaining cognitive function throughout life. Microalgae-derived DHA is increasingly used in infant formula, dietary supplements, and functional foods, providing a vegetarian and sustainable source of this nutrient. The use of microalgal DHA is endorsed by organizations such as the U.S. Food and Drug Administration (FDA), which has granted Generally Recognized as Safe (GRAS) status to several microalgal oil products for use in foods and supplements. This has facilitated the incorporation of DHA into a wide range of consumer products, supporting public health initiatives aimed at improving omega-3 intake.
In the pharmaceutical sector, DHA is being investigated and utilized for its therapeutic potential in managing neurodegenerative diseases, cardiovascular disorders, and inflammatory conditions. The high purity and controlled production conditions of microalgal DHA make it particularly suitable for pharmaceutical applications, where consistency and safety are paramount. Research supported by entities such as the National Institutes of Health (NIH) continues to explore the mechanisms by which DHA exerts its beneficial effects, as well as its potential in novel drug formulations.
Aquaculture represents another major application area for microalgal DHA. As global demand for seafood rises, sustainable aquafeed ingredients are essential to reduce reliance on wild-caught fish for fish oil. Microalgae-based DHA is now incorporated into aquafeeds to enhance the nutritional profile of farmed fish and shrimp, ensuring that these products remain rich sources of omega-3 fatty acids for human consumption. Organizations such as the Food and Agriculture Organization of the United Nations (FAO) recognize the importance of microalgae in advancing sustainable aquaculture practices and reducing pressure on marine ecosystems.
Overall, the biosynthesis of DHA in marine microalgae underpins a rapidly expanding sector that addresses critical needs in nutrition, medicine, and sustainable food production. Continued research and development are expected to further enhance the efficiency and scalability of microalgal DHA production, broadening its impact across multiple industries.
Future Directions and Emerging Research in DHA Biosynthesis
The future of docosahexaenoic acid (DHA) biosynthesis in marine microalgae is shaped by advances in molecular biology, metabolic engineering, and sustainable bioprocessing. As global demand for DHA rises due to its critical role in human health, particularly in neural development and cardiovascular function, research is intensifying to optimize microalgal production systems and uncover novel biosynthetic pathways.
One promising direction involves the genetic engineering of microalgae to enhance DHA yields. By manipulating key enzymes in the polyketide synthase (PKS) and fatty acid synthase (FAS) pathways, researchers aim to redirect metabolic flux toward DHA accumulation. Recent studies have identified regulatory genes and transcription factors that can be targeted to upregulate DHA biosynthesis, while CRISPR/Cas9 and other genome editing tools are being refined for use in diverse microalgal species. These approaches are expected to improve both the efficiency and scalability of DHA production, making it more economically viable for commercial applications.
Another emerging area is the exploration of extremophilic and underexplored marine microalgae. These organisms may possess unique biosynthetic machinery or regulatory mechanisms that confer higher DHA productivity or resilience to environmental stressors. High-throughput screening and omics technologies, such as genomics, transcriptomics, and metabolomics, are being employed to identify and characterize these novel strains. The integration of systems biology with synthetic biology holds promise for the rational design of microalgae with tailored lipid profiles.
Sustainability is a central concern in future research. Efforts are underway to develop closed-loop cultivation systems, utilize non-arable land, and recycle nutrients to minimize the environmental footprint of microalgal DHA production. The use of photobioreactors and optimization of light, temperature, and nutrient regimes are being investigated to maximize productivity while reducing resource inputs. Collaboration between academic institutions, industry, and international organizations is crucial for translating laboratory advances into scalable, sustainable production platforms.
Regulatory and safety considerations are also shaping the research landscape. As microalgal DHA is increasingly incorporated into food, nutraceutical, and pharmaceutical products, ensuring product quality and safety is paramount. Organizations such as the Food and Agriculture Organization of the United Nations and the World Health Organization provide guidelines and standards that inform best practices in cultivation, processing, and product formulation.
In summary, the future of DHA biosynthesis in marine microalgae is marked by interdisciplinary innovation, with advances in genetic engineering, strain discovery, sustainable cultivation, and regulatory frameworks driving the field toward more efficient and responsible production of this essential omega-3 fatty acid.
Sources & References
- World Health Organization
- Food and Agriculture Organization of the United Nations
- Algae Biomass Organization
- National Institute of Genetics
- European Marine Biological Resource Centre
- National Aeronautics and Space Administration (NASA)
- United Nations Environment Programme (UNEP)
- European Food Safety Authority
- National Renewable Energy Laboratory
- Algae Industry Magazine
- National Institutes of Health (NIH)