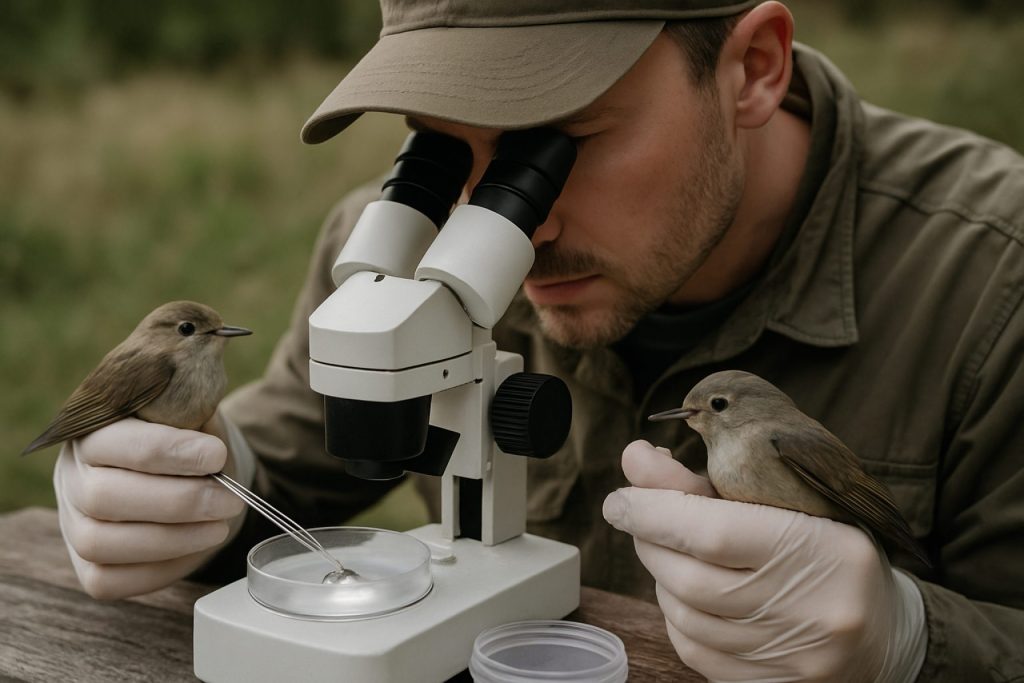
Avian Malaria Vector Surveillance: How Advanced Monitoring is Transforming Our Understanding of Disease Transmission in Bird Populations. Discover the Latest Tools, Trends, and Challenges in Tracking Avian Malaria Vectors.
- Introduction to Avian Malaria and Its Vectors
- Global Distribution and Epidemiology of Avian Malaria
- Key Vector Species: Identification and Biology
- Surveillance Methodologies: Traditional vs. Modern Approaches
- Molecular Tools for Vector Detection and Analysis
- Environmental and Climatic Influences on Vector Populations
- Data Integration and Geospatial Mapping in Surveillance
- Challenges in Field Sampling and Data Collection
- Case Studies: Successful Surveillance Programs Worldwide
- Future Directions and Innovations in Avian Malaria Vector Surveillance
- Sources & References
Introduction to Avian Malaria and Its Vectors
Avian malaria is a parasitic disease caused primarily by protozoa of the genus Plasmodium, which infects a wide range of bird species worldwide. The transmission of avian malaria relies on hematophagous (blood-feeding) insect vectors, predominantly mosquitoes of the family Culicidae. Among these, species within the genera Culex, Aedes, and Anopheles are recognized as the principal vectors responsible for spreading the disease among avian populations. The ecological and epidemiological impact of avian malaria is significant, particularly in regions where native bird species have not co-evolved with the parasite, such as the Hawaiian Islands, leading to severe population declines and even extinctions.
Vector surveillance is a cornerstone of avian malaria research and control. Surveillance involves the systematic collection, identification, and monitoring of mosquito populations to assess their abundance, distribution, and infection rates with Plasmodium parasites. This information is critical for understanding transmission dynamics, identifying high-risk areas, and implementing targeted vector control strategies. Surveillance programs typically employ a combination of field sampling methods, such as light traps, gravid traps, and larval surveys, alongside laboratory-based molecular diagnostics to detect and characterize Plasmodium infections within mosquito populations.
The importance of avian malaria vector surveillance extends beyond academic research. It is essential for the conservation of vulnerable bird species, especially in biodiversity hotspots and isolated ecosystems. For example, in Hawaii, the introduction of Culex quinquefasciatus and subsequent spread of avian malaria have been linked to dramatic declines in native honeycreeper populations. Surveillance data have informed conservation management decisions, such as habitat modification, mosquito control interventions, and the development of disease-resistant bird populations.
Several organizations play a pivotal role in avian malaria vector surveillance. The Centers for Disease Control and Prevention (CDC) provides guidelines and technical support for vector surveillance and control, while the World Health Organization (WHO) offers global leadership in vector-borne disease monitoring and response. In regions with unique conservation challenges, local agencies and research institutions, such as the U.S. Geological Survey (USGS), contribute to long-term surveillance and ecological studies. These collaborative efforts are vital for advancing our understanding of avian malaria transmission and for developing effective strategies to mitigate its impact on avian biodiversity.
Global Distribution and Epidemiology of Avian Malaria
Avian malaria, primarily caused by protozoan parasites of the genus Plasmodium, poses significant threats to wild and domestic bird populations worldwide. The transmission of avian malaria depends on the presence and activity of competent mosquito vectors, predominantly species within the genera Culex, Aedes, and Culiseta. Effective surveillance of these vectors is essential for understanding the global distribution and epidemiology of avian malaria, as well as for informing targeted control strategies.
Vector surveillance involves systematic monitoring of mosquito populations to assess species composition, abundance, seasonal dynamics, and infection rates with avian malaria parasites. Surveillance programs typically employ a combination of trapping methods, such as CDC light traps, gravid traps, and aspirators, to collect adult mosquitoes in habitats frequented by birds. Collected specimens are then identified morphologically or through molecular techniques, and screened for Plasmodium DNA using polymerase chain reaction (PCR) assays. These approaches enable researchers to map the spatial and temporal patterns of vector presence and parasite prevalence, which are critical for predicting disease risk and outbreaks.
Globally, the distribution of avian malaria vectors is influenced by climatic, ecological, and anthropogenic factors. For instance, the spread of Culex quinquefasciatus—a major vector—has been facilitated by urbanization and the creation of artificial water bodies. In regions such as Hawaii, the introduction of non-native mosquito species has led to devastating impacts on endemic bird populations, with avian malaria contributing to the decline and extinction of several species. Surveillance efforts in these sensitive areas are coordinated by governmental and conservation organizations, including the United States Geological Survey and the U.S. Fish and Wildlife Service, which monitor vector populations and disease incidence to guide conservation actions.
Internationally, organizations such as the World Health Organization and the World Organisation for Animal Health (WOAH) provide guidelines and technical support for vector surveillance and disease reporting. These bodies facilitate the sharing of surveillance data and best practices among countries, enhancing the global capacity to detect and respond to emerging avian malaria threats. Additionally, collaborative research networks and academic institutions contribute to the development of innovative surveillance tools, such as remote sensing for habitat mapping and next-generation sequencing for vector and parasite identification.
In summary, avian malaria vector surveillance is a cornerstone of global efforts to understand and mitigate the spread of this disease. By integrating field monitoring, laboratory diagnostics, and international cooperation, surveillance programs provide the data necessary to protect avian biodiversity and ecosystem health.
Key Vector Species: Identification and Biology
Effective avian malaria vector surveillance hinges on the accurate identification and understanding of the biology of key mosquito species responsible for transmitting Plasmodium parasites among bird populations. The primary vectors of avian malaria are mosquitoes in the genera Culex, Aedes, and Mansonia, with Culex pipiens complex being the most significant in temperate regions. These mosquitoes are widely distributed and exhibit a strong preference for avian hosts, making them central to the epidemiology of avian malaria.
Identification of vector species is typically achieved through a combination of morphological analysis and molecular techniques. Morphological identification relies on distinguishing features such as wing patterns, leg banding, and scale arrangements, but this can be challenging due to cryptic species complexes. Molecular methods, including polymerase chain reaction (PCR) and DNA barcoding, have become essential tools for resolving species-level identification, especially within complexes like Culex pipiens and Culex quinquefasciatus. These approaches are recommended by organizations such as the Centers for Disease Control and Prevention and the World Health Organization for their accuracy and reliability in vector surveillance programs.
Understanding the biology of these vectors is crucial for surveillance and control. Key aspects include their breeding habitats, feeding behavior, and seasonal dynamics. Culex mosquitoes typically breed in stagnant water sources such as ponds, marshes, and artificial containers. Their feeding activity is often crepuscular or nocturnal, with a marked preference for avian blood meals, although some species may opportunistically feed on mammals. The life cycle from egg to adult can be completed in as little as one to two weeks under optimal conditions, leading to rapid population increases during warm, wet periods.
Surveillance programs often integrate larval and adult mosquito sampling to monitor vector abundance and distribution. Techniques include the use of light traps, gravid traps, and larval dipping. Collected specimens are then identified and, in some cases, tested for the presence of Plasmodium DNA to assess infection rates. Data from these activities inform risk assessments and guide targeted interventions to reduce transmission.
In summary, the identification and biological characterization of key avian malaria vectors are foundational to effective surveillance. By employing both traditional and molecular identification methods and understanding vector ecology, public health and wildlife agencies can better predict and manage the spread of avian malaria. Leading authorities such as the Centers for Disease Control and Prevention and the World Health Organization provide guidelines and resources to support these efforts globally.
Surveillance Methodologies: Traditional vs. Modern Approaches
Avian malaria, primarily caused by Plasmodium species, poses significant threats to bird populations worldwide, especially in regions where native avifauna have not co-evolved with the parasite. Effective surveillance of avian malaria vectors—mainly mosquitoes of the genera Culex, Aedes, and Anopheles—is crucial for understanding transmission dynamics and implementing control strategies. Surveillance methodologies have evolved from traditional entomological techniques to modern, technology-driven approaches, each with distinct advantages and limitations.
Traditional Surveillance Approaches
Historically, avian malaria vector surveillance has relied on field-based entomological methods. These include the use of light traps, gravid traps, and manual aspirators to collect adult mosquitoes in habitats frequented by birds. Collected specimens are then morphologically identified to species level using taxonomic keys. Blood meal analysis, often through serological assays, helps determine host feeding preferences, while dissection and microscopy are used to detect Plasmodium infections in mosquito tissues. These methods, while foundational, are labor-intensive, require specialized expertise, and may lack sensitivity for detecting low-level infections or cryptic vector species.
Modern Surveillance Approaches
Recent advances have introduced molecular and digital tools that enhance the accuracy and efficiency of avian malaria vector surveillance. Polymerase chain reaction (PCR) and quantitative PCR (qPCR) techniques enable the detection of Plasmodium DNA in mosquito samples, allowing for precise identification of both vector species and parasite lineages. Next-generation sequencing (NGS) further facilitates high-throughput screening of vector populations, uncovering complex host-parasite interactions and cryptic diversity. Geographic Information Systems (GIS) and remote sensing technologies are increasingly used to map vector habitats, model transmission risk, and monitor environmental changes influencing vector distribution. Automated mosquito traps equipped with sensors and real-time data transmission capabilities are also being piloted to streamline field surveillance.
Comparative Assessment and Integration
While traditional methods remain valuable for baseline monitoring and historical comparisons, modern molecular and digital approaches offer greater sensitivity, specificity, and scalability. Integrating both methodologies can provide a comprehensive surveillance framework, combining the ecological context of field observations with the precision of molecular diagnostics. Leading organizations such as the Centers for Disease Control and Prevention and the World Health Organization emphasize the importance of such integrated surveillance systems for vector-borne disease management. Continued innovation and capacity building are essential to address emerging challenges in avian malaria vector surveillance, particularly in the face of climate change and global biodiversity loss.
Molecular Tools for Vector Detection and Analysis
Molecular tools have revolutionized avian malaria vector surveillance by enabling precise detection, identification, and analysis of both mosquito vectors and the Plasmodium parasites they transmit. Traditional surveillance methods, such as morphological identification and dissection, are often labor-intensive and limited in their ability to distinguish closely related species or detect low-level infections. In contrast, molecular techniques offer high sensitivity and specificity, which are critical for understanding vector ecology and disease transmission dynamics.
Polymerase chain reaction (PCR) is the cornerstone of molecular surveillance for avian malaria vectors. PCR-based assays can amplify specific DNA fragments from both the mosquito host and the Plasmodium parasite, allowing for accurate species identification and infection status assessment. For example, mitochondrial cytochrome oxidase I (COI) gene sequencing is widely used for mosquito species barcoding, while nested PCR targeting the Plasmodium cytochrome b gene is commonly employed to detect and differentiate avian malaria parasites. These approaches have been instrumental in uncovering cryptic vector species and mapping their distribution, which is essential for targeted control strategies.
Advancements in real-time quantitative PCR (qPCR) have further enhanced surveillance capabilities by enabling quantification of parasite loads within individual mosquitoes. This information is valuable for assessing vector competence and understanding the epidemiology of avian malaria. Additionally, high-throughput sequencing technologies, such as next-generation sequencing (NGS), allow for comprehensive analysis of mosquito and parasite communities in environmental samples. Metabarcoding approaches can simultaneously identify multiple vector and parasite species from bulk samples, providing a broader picture of transmission networks.
Molecular xenomonitoring, which involves screening mosquito populations for pathogen DNA, is increasingly recognized as a non-invasive and efficient surveillance tool. This method can detect the presence of Plasmodium DNA in mosquito pools, serving as an early warning system for avian malaria outbreaks. The integration of molecular data with geospatial and ecological information supports the development of predictive models for vector distribution and disease risk.
Key organizations such as the Centers for Disease Control and Prevention and the World Health Organization provide guidelines and resources for molecular surveillance of vector-borne diseases, including malaria. Collaborative networks, such as the European Centre for Disease Prevention and Control, also contribute to standardizing protocols and sharing data across regions. These efforts are vital for harmonizing surveillance activities and informing public health interventions aimed at mitigating the impact of avian malaria on wildlife and, potentially, human populations.
Environmental and Climatic Influences on Vector Populations
Environmental and climatic factors play a pivotal role in shaping the distribution, abundance, and activity of mosquito vectors responsible for avian malaria transmission. Surveillance of these vectors—primarily mosquitoes of the genera Culex, Aedes, and Anopheles—requires a nuanced understanding of how ecological variables and climate dynamics influence their populations and, consequently, the risk of avian malaria outbreaks.
Temperature is a critical determinant of mosquito development rates, survival, and vector competence. Warmer temperatures can accelerate the life cycle of mosquitoes, increase biting frequency, and shorten the extrinsic incubation period of Plasmodium parasites within the vector. Conversely, extreme heat or cold can reduce mosquito survival, thereby impacting transmission potential. Seasonal temperature fluctuations often dictate the timing and intensity of vector surveillance efforts, with heightened monitoring during warmer months when mosquito activity peaks.
Precipitation patterns also significantly affect mosquito breeding habitats. Rainfall creates standing water, which serves as larval habitats for many vector species. However, excessive rainfall can flush out breeding sites, while drought conditions may limit available habitats. Surveillance programs must therefore adapt to local hydrological conditions, employing larval and adult mosquito sampling techniques that reflect current environmental realities. The use of remote sensing and geographic information systems (GIS) has become increasingly important for mapping potential breeding sites and predicting vector hotspots based on rainfall and land cover data.
Habitat characteristics, such as the presence of wetlands, forested areas, and urban environments, further influence vector populations. For example, Culex species often thrive in stagnant water bodies commonly found in both natural and artificial settings. Land use changes, including deforestation and urbanization, can alter mosquito community composition and increase contact rates between vectors and avian hosts. Surveillance strategies must therefore be tailored to specific ecological contexts, integrating habitat assessments with entomological monitoring.
Climate change is expected to modify the geographic range and seasonality of avian malaria vectors, potentially introducing the disease to new regions and avian populations. Long-term surveillance data are essential for detecting such shifts and informing adaptive management strategies. International organizations such as the World Health Organization and national agencies like the Centers for Disease Control and Prevention provide guidelines and technical support for vector surveillance, emphasizing the integration of environmental and climatic data into risk assessment frameworks.
In summary, effective avian malaria vector surveillance hinges on a comprehensive understanding of environmental and climatic influences. By incorporating ecological monitoring, climate modeling, and advanced geospatial tools, surveillance programs can better anticipate changes in vector populations and mitigate the risks posed to avian biodiversity.
Data Integration and Geospatial Mapping in Surveillance
Effective avian malaria vector surveillance increasingly relies on the integration of diverse data sources and advanced geospatial mapping techniques. The primary vectors of avian malaria, such as mosquitoes of the genus Culex, are distributed across varied ecological landscapes, making spatially explicit data crucial for understanding transmission dynamics and informing control strategies. Integrating entomological, environmental, and avian host data enables researchers to identify vector hotspots, track changes in vector populations, and predict areas at risk for disease emergence.
Data integration in avian malaria surveillance involves the collection and harmonization of information from multiple domains. Entomological data, including mosquito species identification, abundance, and infection rates, are combined with avian host distribution and infection prevalence. Environmental variables—such as temperature, precipitation, land cover, and water body proximity—are also incorporated, as these factors influence both vector and host populations. The use of standardized data collection protocols and interoperable databases is essential for ensuring data quality and comparability across regions and time periods. Organizations like the Centers for Disease Control and Prevention (CDC) and the World Health Organization (WHO) provide guidelines and resources for vector surveillance and data management.
Geospatial mapping tools, including Geographic Information Systems (GIS) and remote sensing technologies, play a pivotal role in visualizing and analyzing the spatial distribution of vectors and disease risk. GIS platforms allow researchers to overlay vector and host data with environmental layers, facilitating the identification of spatial patterns and potential drivers of transmission. Remote sensing data, such as satellite-derived land cover and climate variables, enhance the ability to monitor environmental changes that may affect vector habitats. These technologies support the development of risk maps, which are instrumental for targeting surveillance and control efforts in high-risk areas.
Collaborative initiatives, such as those led by the World Organisation for Animal Health (WOAH, formerly OIE), promote the sharing of surveillance data and best practices among countries and regions. Such cooperation is vital for tracking the spread of avian malaria vectors, particularly in the context of global change and the movement of migratory birds. The integration of data and geospatial mapping not only enhances the efficiency of surveillance programs but also supports evidence-based decision-making for vector control and avian health management.
Challenges in Field Sampling and Data Collection
Avian malaria vector surveillance is essential for understanding the transmission dynamics of avian malaria and for implementing effective control strategies. However, field sampling and data collection in this context present several significant challenges that can impact the accuracy and reliability of surveillance efforts.
One of the primary challenges is the diversity and behavior of mosquito vectors. Avian malaria is primarily transmitted by mosquitoes in the genera Culex, Aedes, and Mansonia, which often exhibit varied habitat preferences and feeding behaviors. This diversity necessitates the use of multiple sampling methods, such as light traps, gravid traps, and aspirators, to capture a representative sample of vector populations. However, each method has inherent biases and limitations, potentially leading to underrepresentation of certain species or life stages (Centers for Disease Control and Prevention).
Environmental factors further complicate field sampling. Mosquito abundance and activity are highly influenced by climatic conditions, habitat type, and seasonality. In remote or densely vegetated areas, accessibility can be limited, making it difficult to deploy and retrieve traps consistently. Additionally, weather events such as heavy rainfall or drought can alter mosquito breeding sites, leading to temporal fluctuations in vector populations and complicating longitudinal studies (World Health Organization).
Another challenge is the identification of mosquito species and the detection of avian malaria parasites within vectors. Morphological identification requires specialized expertise, as many vector species are morphologically similar. Molecular techniques, such as PCR, offer higher specificity but require laboratory infrastructure, trained personnel, and resources that may not be available in all field settings. The preservation and transport of samples from remote locations to laboratories can also affect the quality and integrity of specimens, potentially leading to data loss or misidentification.
Data collection is further hindered by logistical and resource constraints. Field surveillance often requires significant manpower, funding, and coordination, especially when conducted over large geographic areas or extended time periods. In many regions, there is a lack of standardized protocols for sampling and data reporting, which can result in inconsistent data and hinder comparisons across studies or regions (World Organisation for Animal Health).
In summary, avian malaria vector surveillance faces multifaceted challenges in field sampling and data collection, stemming from vector diversity, environmental variability, technical limitations, and logistical constraints. Addressing these challenges is critical for improving surveillance accuracy and informing effective disease management strategies.
Case Studies: Successful Surveillance Programs Worldwide
Avian malaria, primarily caused by Plasmodium species, poses a significant threat to bird populations worldwide, especially in regions where native avifauna have not co-evolved with the parasite. Effective surveillance of mosquito vectors is crucial for early detection, risk assessment, and the implementation of control strategies. Several countries and organizations have established successful avian malaria vector surveillance programs, providing valuable models for global efforts.
One of the most renowned case studies is from Hawaii, where the introduction of Culex quinquefasciatus mosquitoes in the 19th century led to devastating declines in native bird species. The United States Geological Survey (USGS) has played a pivotal role in long-term vector surveillance on the Hawaiian Islands. Their integrated approach combines mosquito trapping, molecular identification of Plasmodium in both vectors and avian hosts, and environmental monitoring. This program has enabled researchers to map vector distribution, track seasonal fluctuations, and correlate mosquito abundance with avian malaria outbreaks. The data have informed targeted vector control and habitat management, contributing to the conservation of endangered Hawaiian honeycreepers.
In Europe, the European Centre for Disease Prevention and Control (ECDC) coordinates vector surveillance across member states, including monitoring for avian malaria vectors such as Culex pipiens and Culex modestus. National public health institutes, in collaboration with ornithological societies, conduct regular mosquito sampling in wetlands and urban areas. Molecular diagnostics are used to detect Plasmodium DNA in captured mosquitoes, providing early warning of potential transmission hotspots. The ECDC’s harmonized protocols facilitate data sharing and rapid response to emerging threats, exemplifying the benefits of cross-border collaboration.
In South America, Brazil’s Ministry of Health, in partnership with academic institutions, has implemented surveillance programs in the Amazon and Atlantic Forest regions. These initiatives focus on both vector and avian host sampling, using a combination of light traps, larval surveys, and blood meal analysis. The integration of ecological and epidemiological data has improved understanding of vector ecology and the impact of environmental changes on disease dynamics.
These case studies highlight the importance of multi-disciplinary collaboration, standardized methodologies, and the use of molecular tools in avian malaria vector surveillance. Organizations such as the World Health Organization (WHO) and regional public health agencies continue to promote best practices and capacity building, ensuring that surveillance programs remain robust and responsive to emerging challenges.
Future Directions and Innovations in Avian Malaria Vector Surveillance
The future of avian malaria vector surveillance is poised for significant transformation, driven by technological advancements, interdisciplinary collaboration, and a growing recognition of the ecological and public health implications of avian malaria. Traditional surveillance methods, such as field sampling and morphological identification of mosquito vectors, are increasingly being complemented and, in some cases, replaced by innovative approaches that enhance detection accuracy, efficiency, and scalability.
One of the most promising directions is the integration of molecular techniques, such as polymerase chain reaction (PCR) and next-generation sequencing (NGS), into routine surveillance. These methods allow for the rapid and precise identification of both mosquito species and the presence of Plasmodium parasites, even at low infection rates. Molecular barcoding and metagenomic analyses can uncover cryptic vector species and track the spread of parasite lineages across regions, providing a more comprehensive understanding of transmission dynamics. Organizations like the Centers for Disease Control and Prevention and the World Health Organization have highlighted the importance of molecular diagnostics in vector-borne disease surveillance.
Remote sensing and geographic information systems (GIS) are also revolutionizing avian malaria vector surveillance. By leveraging satellite imagery and environmental data, researchers can model mosquito habitat suitability, predict vector population dynamics, and identify potential hotspots for disease transmission. These spatial tools enable targeted surveillance and intervention strategies, optimizing resource allocation and improving early warning systems. The National Aeronautics and Space Administration and the Food and Agriculture Organization of the United Nations are among the entities advancing the use of remote sensing for vector monitoring.
Another innovative frontier is the deployment of automated mosquito traps equipped with artificial intelligence (AI) and machine learning algorithms. These smart traps can autonomously identify mosquito species based on wingbeat frequency, morphology, or genetic markers, transmitting real-time data to centralized databases. Such automation reduces labor costs and human error, while enabling continuous, large-scale surveillance. Collaborative efforts between academic institutions, governmental agencies, and technology companies are accelerating the development and field-testing of these systems.
Looking ahead, the integration of citizen science platforms and mobile applications may further democratize avian malaria vector surveillance. Engaging local communities in data collection and reporting can expand surveillance coverage, especially in remote or resource-limited areas. As climate change and global trade alter vector distributions, adaptive and innovative surveillance systems will be essential for mitigating the impacts of avian malaria on wildlife, ecosystems, and, potentially, human health.
Sources & References
- Centers for Disease Control and Prevention
- World Health Organization
- U.S. Fish and Wildlife Service
- European Centre for Disease Prevention and Control
- National Aeronautics and Space Administration
- Food and Agriculture Organization of the United Nations